Role of the anterolateral complex in rotatory instability of the anterior cruciate ligament deficient knee
Introduction
Injuries to the anterior cruciate ligament (ACL) alter the kinematics of the tibiofemoral joint significantly. Failure to restore the native knee biomechanics can cause long-term functional impairment and degenerative changes (1,2). The goals of ACL reconstruction are to re-establish the physiological anterior translation and internal rotation of the tibia relative to the femur without over-constraining the knee joint.
Rotatory knee laxity plays a crucial role in ACL injuries and treatment algorithms (3). Increased rotatory knee laxity, assessed most commonly with the pivot shift test, indicates damage to the ACL and anterolateral knee structures (4). Although the clinical results of the ACL reconstruction are usually excellent (5), there is a subgroup of patients that continues to experience residual rotatory instability. This rotatory laxity is a matter of concern among surgeons, who ascribe this failure to overlooked associated injuries, different bony morphologic patterns, or inadequate reproduction of the native ACL anatomical footprints (6). This has led physicians to seek out other causes and treatments of anterolateral rotatory knee laxity.
The ACL is considered the primary restraint to anterior and rotatory knee stability, including both internal and external rotation, but there are many secondary stabilizers that play an important role. Notably, the posterior horn of the medial meniscus and the lateral meniscus provide an important contribution. Additionally, the anterolateral structures of the knee contribute to rotatory knee stability (7). Recently, a ligamentous structure in the lateral aspect of the knee called the anterolateral ligament (ALL) has been described. Some authors advocated its role in supplementing the ACL in controlling rotational laxity and internal rotation (8).
Additional stabilizing procedures during ACL reconstruction have emerged to address this residual rotatory laxity. These lateral extra-articular tenodesis (EAT) procedures have been biomechanically shown to decrease internal tibial rotation; conversely, they have also been shown to over-constrain the knee (9). Recently, anatomic ALL-specific reconstructions have emerged with conflicting results.
These contrasting findings of the ALL, and emergence of supplemental soft tissue procedures, have triggered a debate about its precise anatomical position, its biomechanical role and the need for additional surgical procedures.
Anatomy
In the earliest accounts of the ALL, Paul Segond in 1879 described a “pearly, resistant, fibrous band” on the anterolateral aspect of the knee that caused an avulsion fracture during forced knee internal rotation, now known as the “Segond fracture” (10). However, this structure was not mentioned again in anatomic descriptions or textbooks for nearly another century.
Ambiguous anatomic descriptions of this capsular thickening ensued, including “mid-third lateral capsular ligament” (11-13), “capsule-osseous layer of the iliotibial band (ITB)” (14,15), and “anterior oblique band” (16). Recently, cadaveric studies have expanded on this work and anatomically described the anterolateral structures of the knee, including this so-called “ALL” (14,15,17).
In the study by Claes et al. (17), the ALL was described as a distinct ligamentous structure at the anterolateral side of the knee joint, clearly distinguishable from the capsule, the ITB, and the lateral collateral ligament (LCL). Since this study, various attempts have been made to localize the femoral and tibial insertion of the ALL as well as its fiber orientation, adhesion to the capsule and the lateral meniscus.
Multiple attachment sites of the proximal insertion site of the ALL have since been described. Claes et al. (17) described a structure that originates from the lateral femoral epicondyle, posterior and proximal to the insertion of the popliteus, and anterior to the femoral insertion of the LCL. Soon after this, a study utilizing fresh-frozen cadavers demonstrated the femoral attachment site to be 9 mm proximal to the lateral epicondyle (18). Another study detailed the femoral attachment to be 3.5 mm distal and 2.2 mm anterior to the attachment of the LCL (19).
Most authors agree that the distal insertion is located midway between the center of Gerdy’s tubercle and the anterior margin of the fibular head, approximately 4.5 mm from the most distal articular portion of the tibia (17-19).
Other ongoing concerns with the ALL are its relation with the knee joint capsule and the lateral meniscus. Some authors (17,18,20) describe the ALL as a ligament that is distinguishable from the capsule, while others (21-23) denote the ALL is unequivocally a thickening of the knee joint capsule.
Additionally, histological analysis of the ALL remains an unresolved and controversial issue. Vincent et al. (24) documented wavy collagenous fibers with a parallel orientation in the core of the capsule, suggesting the presence of a distinct ligamentous structure. However, Dombrowski et al. (25) did identify capsular thickening in only 30% of specimens with “ligament-like” characteristics.
ALL prevalence
Although in some cadaveric studies the ALL was identified in 80–100% of the specimens (17-19,23,24), other investigators have reported it is possible to clearly identify it in only 30–50% of the samples (20,22,26). Additionally, a recent study reported that none of the specimens showed a clear lateral capsular ligament (25) (Figure 1).
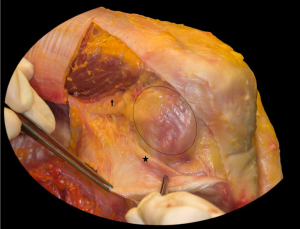
This ambiguity in anatomic findings may be due to various specimen preservation techniques, a wide age range of the specimens, different anatomic dissection techniques, and inconsistent terminology. The cadaveric studies utilized different methods of preservation including embalmed and fresh-frozen specimens, which can alter the integrity of small structures, especially in the anterolateral complex (ALC). Interestingly, some authors have highlighted the discovery of the ALL with their dissection techniques by internally rotating the tibia and removing any surrounding tissue without tension (23,27). With such ambiguity, the anterolateral aspect of the knee, including the ALL, ITB, and capsulo-osseous layer of the ITB, will be collectively defined as the ALC (Figure 2). Consequently, biomechanical studies have suggested all the structures of the ALC contribute to rotatory knee stability as a whole unit, rather than as individual ligaments (28,29), further confirming the complex as a collective unit.
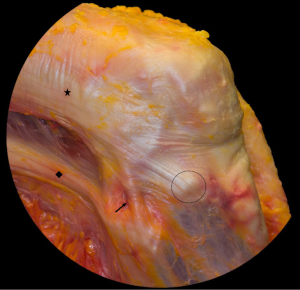
Imaging
Proper imaging modalities to diagnose injury to the ACL and concomitant lateral structures are paramount for treatment purposes and patient outcomes. Anatomy of these structures has been well documented throughout the years, with the only current controversy regarding the existence of an ALL. While the diagnosis of ACL injuries has been well described and implemented, radiographic determination of ALC injuries is more complex and controversial. To date, imaging modalities to detect ALC injuries include x-ray, ultrasound, and magnetic resonance imaging (MRI).
X-ray
Highly regarded as a pathognomonic sign of ACL injury, the Segond fracture is a cortical avulsion off the anterolateral aspect of the tibia seen in around 1% of ACL injuries. This fracture results from excessive internal rotation and varus torque to the knee (10). This fleck of bone can be visualized an AP views of the knee. In 1979, Woods et al. (30) suggested this fleck of bone represented an avulsion fracture of the lateral capsular ligament, indicating a high-grade ACL injury. The authors also postulated this fracture represented a repairable injury to the lateral knee capsule and proceeded with EAT on all patients who presented with this fracture (30). Other studies validated this finding, concluding the Segond fracture was in fact an avulsion of the lateral capsular ligament and represented injury to the ACL (31,32). Most recently, this avulsion fracture has been intimately associated with the ALL (33). Although the exact etiology of the Segond fracture remains controversial, studies concur this avulsion fracture represents a rotatory knee injury mechanism and ACL tear.
Ultrasound
Ultrasound can be a powerful and relatively inexpensive imaging modality to assess the supporting structures of the knee. Although the knee anatomy is complex, ultrasound has been demonstrated to produce an accurate and detailed evaluation of knee anatomic structures, including the lateral knee complex (34). However, conflicting studies have emerged describing the ability of ultrasound to detect the ALL.
Although challenging, one study demonstrated the ability to distinguish its femoral and tibial attachment sites when the knee was brought into flexion and internal rotation (35). A recent cadaveric study found the tibial portion of the ALL could be distinguished on all specimens, but the meniscal and femoral attachments could only be identified in <50% of the specimens (36). Another study utilized real-time virtual ultrasonography and visualized a distinguishable ALL in 7 of 9 subjects (36).
On the contrary, ultrasound was utilized on ten cadaveric knees and found a large discrepancy between the ultrasonographic and dissection findings. The authors found a mean ALL length on ultrasound and dissection to be 10.9 mm (range, 7.9–15.8 mm) and 12.5 mm (range, 3.2–19.3 mm), respectively. They concluded ultrasound was unable to reliably identify the ALL attachment sites, and it is possible that ALL could be a thickened band of fascia rather than a true ligament. Additionally, they stated that distinguishing the ALL from the posterior ITB and the anterolateral capsule was challenging (37). Another study utilized ultrasound to identify, in great detail, the lateral structures of the knee, but failed to identify an ALL in any subject (38). In summary, ultrasound can be a useful and cheap alternative to detect injury to the ALC, but distinguishing injury to individual structures is more problematic and difficult.
MRI
MRIs are routinely obtained on patients with a suspected ACL injury, and are highly sensitive and specific for an ACL tear (39). However, the complexity of the ALC, and specifically identifying individual structures such as the ALL, can decrease the utility of MRI.
Some have reported that MRI yields accurate visualization of all lateral knee structures, including the ITB and LCL (12,16). A retrospective review of ACL injuries demonstrated 100% visualization of the ALL, but poor intra-observer and inter-observer reliability in assessing injuries to the ALL. They concluded MRI has poor reliability in distinguishing between a torn and intact ALL (40). Another group compared MRI results with cadaveric specimens, and was able to visualize the ALL in all specimens (41).
Conversely, a separate study utilizing cadavers to compare anatomic findings with MRI findings demonstrated variable thickness of the lateral capsule without a discernable ligamentous structure (25). A fresh-frozen cadaveric study revealed morphologic variations in the ALC anatomy, with the inability to detect an ALL (25). A study from our own research group attempted to identify and grade ALC injuries in ACL-injured patients, but found only 51% of patients had a discrete injury to their ALC and less than 4% had complete disruption (42). Overall, MRI does have a utility in detecting injuries to the ALC, but delineating injuries to specific structures will require further research.
Rotatory knee stability
Rotatory knee stability involves the combined efforts of soft tissue tensioning and bony morphology. Recently, biomechanical studies have demonstrated the importance of the ALC on internal tibial rotatory knee stability, and have attempted to describe the various roles of the individual components. The ITB has been shown to make a significant contribution, especially at higher flexion angles. Tibial internal rotation is increased with sectioning of the Kaplan fibers and capsulo-osseous layer (43). There is little contribution of the ALL, with no increase in tibial internal rotation after sectioning of the ALL with an intact ITB (43).
Several robotic studies have found an increase in tibia internal rotation in ALL-deficient knees, with the greatest difference seen at 60 degrees of knee flexion (44,45). However, during a simulated pivot shift test, one study only found a small difference in tibia internal rotation after the ALL was sectioned (44); a similar study demonstrated a maximum difference of 3.3 degrees with tibial internal rotation at 45 degrees of knee flexion between ALL-deficient and ALL-intact knees. At lower knee flexion angles where the clinical pivot shift occurs, the contribution of the ALL became negligible (46). These studies indicate the ALC, especially the ITB, contributes to rotatory knee stability, but the role of the ALL can be considered minor.
Pivot shift
The pivot shift test assesses the complex kinematic motions of the knee, including anterolateral rotatory laxity, and is the most specific test for detecting ACL injury (Figure 3) (48). Several studies have indicated that the degree of pivot shift is correlated with return to play, patient satisfaction, overall knee function, and subjective knee stability after ACL reconstruction (2,49). As such, the goal of ACL reconstruction is to restore the normal biomechanics of the knee and minimize or eliminate the rotatory knee laxity that leads to a pivot shift. However, multiple variables contribute to this complex motion, including the ACL, capsule, lateral and medial menisci, bony morphology, and ligamentous knee laxity (50-54).
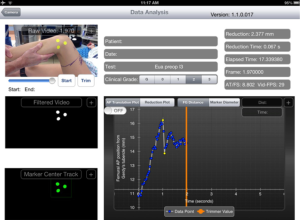
As described earlier, the ALC has been shown to provide secondary restraint to knee internal rotation and rotatory knee stability. Acute injuries of the ALC, as seen on MR images, were shown to be risk factors for high grade pivot shift (42,55). A recent biomechanical cadaveric study indicated the influence of the ALC on knee internal rotation and rotatory knee laxity increases as knee flexion increases beyond 30 degrees (28).
However, controversy still remains regarding the influence of the ALL versus the entire ALC. One study demonstrated the influence of the ALL on internal rotation at flexion angles greater than 30 degrees exceeded the contribution of the ACL (45). The ALL was also shown to be an important secondary stabilizer to knee internal rotation and rotatory knee laxity during knee flexion in ACL-deficient knees (46,56,57). Conversely, a cadaveric biomechanical study determined lesions of the ALL with an intact ITB did not influence rotatory knee laxity in an ACL-deficient knee (58). Another study showed the ALL played only a minor role in restraining internal rotation during knee flexion, while the ITB was the major secondary stabilizer to internal rotation at 30 and 90 degrees of knee flexion (43).
The prior studies demonstrated contributions from the ALL at knee flexion angles greater than 30 degrees. The clinical pivot shift tests rotatory knee stability at knee flexion angles less than 30 degrees. Therefore, the contribution of the ALC to the clinical pivot shift remains unclear. In fact, one study described negligible contributions from the ALL beyond the physiologic limits of motion of the knee (59). Another study demonstrated that at 30 degrees of knee flexion, there was no difference in the in-situ forces between the ACL and ALC during a simulated pivot shift test, indicating the ALC plays a limited role in the clinical pivot shift (28). A recent clinical study even demonstrated an 8.4% residual pivot shift after combined ACL and ALL reconstruction (60), suggesting that other soft tissue restraints besides the ALL and ACL may be present at physiologic ranges of tibial translation. As such, surgeons should utilize this knowledge when contemplating surgical options after an ACL tear, especially additional soft-tissue procedures.
Surgical options
The concept of surgical fixation to control anterolateral knee rotation with EAT procedures has been present for decades. In the 1970’s, surgeons utilized various EAT procedures as the sole surgical operation to address ACL-deficient knees (61). However, two landmark studies emerged in the late 1970’s that demonstrated poor long-term outcomes with EAT alone for ACL-deficient knees (62,63). It was determined the grafts stretched out and only provided temporary stability. As such, the paradigm shifted to intra-articular ACL reconstruction alone, especially after studies emerged demonstrating no difference in functional outcomes between ACL reconstruction and ACL reconstruction with the addition of EAT procedures (64).
Lateral EAT
Recently, renewed interest in EAT procedures have emerged in order to address injury to the ALC and improve rotatory control of the knee (65). Initial studies produced promising results. A cadaveric biomechanical study demonstrated EAT significantly decreased the forces on the ACL by 43% (66). The addition of EAT to ACL reconstruction can be an effective procedure, and showed minimal complications at 2-year follow-up (60). Marcacci et al. reported long-term outcomes on patients treated with hamstring autograft ACL reconstruction and lateral tenodesis. They demonstrated only 2 (out of 54) patients had greater than 5 mm side-to-side difference in anterior-posterior laxity, and 90% of the patients having good or excellent IKDC scores (67). A long-term follow-up study determined the addition of EAT had improved knee stability, no increased risk of osteoarthritis, and a decreased rate of ACL failure (68). Other studies have demonstrated no benefit to the addition of EAT. A recent prospective, randomized control trial demonstrated no significant benefits to patient outcomes with the addition of EAT to ACL reconstruction (69).
ALL reconstruction
As the renewed interest in the ALL emerged, numerous studies appeared that focused on anatomic ALL reconstruction. Due to the multiple anatomic ALL configurations documented, one study attempted to standardize the anatomic landmarks by utilizing cadaveric specimens to document radiographic landmarks for ALL reconstruction (70). A robotic cadaveric study demonstrated anatomic ALL reconstruction significantly improved rotatory knee instability after ACL reconstruction (44). On the other hand, a separate study demonstrated anatomic ALL reconstruction did not reduce rotational knee laxity. This study suggested the ALL becomes functional at unphysiologic knee motions and only engages the tibia once tibial displacement extends beyond the physiologic knee motion of an ACL-intact knee. The authors concluded that EAT procedures may diminish anterior and rotational knee laxity, but ALL reconstruction is not necessarily needed in a well-functioning knee (56).
It is important to note that both EAT procedures and ALL reconstruction have been demonstrated to potentially over-constrain the knee. A cadaveric study concluded ALL augmentation significantly reduced rotatory knee laxity, but resulted in over-constraint of the knee at all flexion angles (9). The addition of EAT has also been shown to cause excess external rotation as compared to normal or ACL reconstructed knees (66), potentially leading to unfavorable long-term outcomes.
Conclusions
The ALC, which includes the ITB, with its deep and capsulo-osseous layer, Kaplan fibers, lateral capsule, and mid third capsular ligament plays an important role in rotatory knee stability. After an ACL injury, recognition of concurrent injuries to the ALC of the knee is important to assess rotatory knee instability. Apart from soft tissue restraints, factors such as bone morphology, muscular control, proprioception and hypermobility have an impact on rotatory knee stability. It is, therefore, crucial to consider all aspects and offer a tailored surgery to each individual patient.
Recently described surgical approaches to address ALC injuries are still controversial with conflicting results. Although lacking evidence in larger patient cohorts, these additional surgical procedures may be useful in a selected subset of patients. Future research into these procedures is warranted, along with an individualized approach to patients with ALC injuries and injuries to the ACL.
Acknowledgments
Funding: None.
Footnote
Provenance and Peer Review: This article was commissioned by the editorial office, Annals of Joint for the series “Trends in ACL Reconstruction”. The article has undergone external peer review.
Conflicts of Interest: All authors have completed the ICMJE uniform disclosure form (available at http://dx.doi.org/10.21037/aoj.2017.06.09). The series “Trends in ACL Reconstruction” was commissioned by the editorial office without any funding or sponsorship. JMB served as the unpaid Guest Editor of the series. The authors have no other conflicts of interest to declare.
Ethical Statement: The authors are accountable for all aspects of the work in ensuring that questions related to the accuracy or integrity of any part of the work are appropriately investigated and resolved.
Open Access Statement: This is an Open Access article distributed in accordance with the Creative Commons Attribution-NonCommercial-NoDerivs 4.0 International License (CC BY-NC-ND 4.0), which permits the non-commercial replication and distribution of the article with the strict proviso that no changes or edits are made and the original work is properly cited (including links to both the formal publication through the relevant DOI and the license). See: https://creativecommons.org/licenses/by-nc-nd/4.0/.
References
- Jonsson H, Riklund-Ahlstrom K, Lind J. Positive pivot shift after ACL reconstruction predicts later osteoarthrosis: 63 patients followed 5-9 years after surgery. Acta Orthop Scand 2004;75:594-9. [Crossref] [PubMed]
- Kocher MS, Steadman JR, Briggs KK, et al. Relationships between objective assessment of ligament stability and subjective assessment of symptoms and function after anterior cruciate ligament reconstruction. Am J Sports Med 2004;32:629-34. [Crossref] [PubMed]
- Musahl V, Kopf S, Rabuck S, et al. Rotatory knee laxity tests and the pivot shift as tools for ACL treatment algorithm. Knee Surg Sports Traumatol Arthrosc 2012;20:793-800. [Crossref] [PubMed]
- Losee RE. Concepts of the pivot shift. Clin Orthop Relat Res 1983;45-51. [PubMed]
- Samitier G, Marcano AI, Alentorn-Geli E, et al. Failure of Anterior Cruciate Ligament Reconstruction. Arch Bone Jt Surg 2015;3:220-40. [PubMed]
- Group M. Descriptive epidemiology of the Multicenter ACL Revision Study (MARS) cohort. Am J Sports Med 2010;38:1979-86. [Crossref] [PubMed]
- Wroble RR, Grood ES, Cummings JS, et al. The role of the lateral extraarticular restraints in the anterior cruciate ligament-deficient knee. Am J Sports Med 1993;21:257-62; discussion 63. [Crossref] [PubMed]
- Dodds AL, Gupte CM, Neyret P, et al. Extra-articular techniques in anterior cruciate ligament reconstruction: a literature review. J Bone Joint Surg Br 2011;93:1440-8. [Crossref] [PubMed]
- Schon JM, Moatshe G, Brady AW, et al. Anatomic Anterolateral Ligament Reconstruction of the Knee Leads to Overconstraint at Any Fixation Angle. Am J Sports Med 2016;44:2546-56. [Crossref] [PubMed]
- Segond P. Recherches cliniques et expérimentales sur les épanchements sanguins du genou par entorse. Paris: Aux bureaux du Progrès médical; 1879.
- Hughston JC, Andrews J, Cross M, et al. Classification of knee ligament instabilities. Part II. The lateral compartment. J Bone Joint Surg Am 1976;58:173-9. [Crossref] [PubMed]
- LaPrade RF, Gilbert TJ, Bollom TS, et al. The Magnetic Resonance Imaging Appearance of Individual Structures of the Posterolateral Knee A Prospective Study of Normal Knees and Knees with Surgically Verified Grade III Injuries. Am J Sports Med 2000;28:191-9. [PubMed]
- Haims AH, Medvecky MJ, Pavlovich R Jr, et al. MR imaging of the anatomy of and injuries to the lateral and posterolateral aspects of the knee. AJR Am J Roentgenol 2003;180:647-53. [Crossref] [PubMed]
- Vieira EL, Vieira EA, da Silva RT, et al. An anatomic study of the iliotibial tract. Arthroscopy 2007;23:269-74. [Crossref] [PubMed]
- Terry GC, Hughston JC, Norwood LA. The anatomy of the iliopatellar band and iliotibial tract. Am J Sports Med 1986;14:39-45. [Crossref] [PubMed]
- Campos JC, Chung CB, Lektrakul N, et al. Pathogenesis of the Segond Fracture: Anatomic and MR Imaging Evidence of an Iliotibial Tract or Anterior Oblique Band Avulsion 1. Radiology 2001;219:381-6. [Crossref] [PubMed]
- Claes S, Vereecke E, Maes M, et al. Anatomy of the anterolateral ligament of the knee. J Anat 2013;223:321-8. [Crossref] [PubMed]
- Dodds AL, Halewood C, Gupte CM, et al. The anterolateral ligament: Anatomy, length changes and association with the Segond fracture. Bone Joint J 2014;96-B:325-31. [Crossref] [PubMed]
- Helito CP, Demange MK, Bonadio MB, et al. Anatomy and Histology of the Knee Anterolateral Ligament. Orthop J Sports Med 2013;1:2325967113513546 [Crossref] [PubMed]
- Runer A, Birkmaier S, Pamminger M, et al. The anterolateral ligament of the knee: A dissection study. Knee 2016;23:8-12. [Crossref] [PubMed]
- Kennedy MI, Claes S, Fuso FA, et al. The Anterolateral Ligament: An Anatomic, Radiographic, and Biomechanical Analysis. Am J Sports Med 2015;43:1606-15. [Crossref] [PubMed]
- Stijak L, Bumbasirevic M, Radonjic V, et al. Anatomic description of the anterolateral ligament of the knee. Knee Surg Sports Traumatol Arthrosc 2016;24:2083-8. [Crossref] [PubMed]
- Caterine S, Litchfield R, Johnson M, et al. A cadaveric study of the anterolateral ligament: re-introducing the lateral capsular ligament. Knee Surg Sports Traumatol Arthrosc 2015;23:3186-95. [Crossref] [PubMed]
- Vincent JP, Magnussen RA, Gezmez F, et al. The anterolateral ligament of the human knee: an anatomic and histologic study. Knee Surg Sports Traumatol Arthrosc 2012;20:147-52. [Crossref] [PubMed]
- Dombrowski ME, Costello JM, Ohashi B, et al. Macroscopic anatomical, histological and magnetic resonance imaging correlation of the lateral capsule of the knee. Knee Surg Sports Traumatol Arthrosc 2016;24:2854-60. [Crossref] [PubMed]
- Watanabe J, Suzuki D, Mizoguchi S, et al. The anterolateral ligament in a Japanese population: Study on prevalence and morphology. J Orthop Sci 2016;21:647-51. [Crossref] [PubMed]
- Kosy JD, Soni A, Venkatesh R, et al. The anterolateral ligament of the knee: unwrapping the enigma. Anatomical study and comparison to previous reports. J Orthop Traumatol 2016;17:303-308. [Crossref] [PubMed]
- Bell KM, Rahnemai-Azar AA, Irarrazaval S, et al. In-Situ Forces in the Anterolateral Capsule Resulting from a Simulated Pivot Shift Test. Orthop Res Soc 2016; Poster No. 126.
- Guenther D, Rahnemai-Azar AA, Bell K, et al. The Anterolateral Capsule of the Knee Behaves Like a Sheet of Fibrous Tissue. Am J Sports Med 2017;45:849-55. [Crossref] [PubMed]
- Woods GW, Stanley RF, Tullos HS. Lateral capsular sign: x-ray clue to a significant knee instability. Am J Sports Med 1979;7:27-33. [Crossref] [PubMed]
- Dietz GW, Wilcox DM, Montgomery JB. Segond tibial condyle fracture: lateral capsular ligament avulsion. Radiology 1986;159:467-9. [Crossref] [PubMed]
- Goldman AB, Pavlov H, Rubenstein D. The Segond fracture of the proximal tibia: a small avulsion that reflects major ligamentous damage. AJR Am J Roentgenol 1988;151:1163-7. [Crossref] [PubMed]
- Claes S, Luyckx T, Vereecke E, et al. The Segond fracture: a bony injury of the anterolateral ligament of the knee. Arthroscopy 2014;30:1475-82. [Crossref] [PubMed]
- De Maeseneer M, Marcelis S, Boulet C, et al. Ultrasound of the knee with emphasis on the detailed anatomy of anterior, medial, and lateral structures. Skeletal Radiol 2014;43:1025-39. [PubMed]
- Cianca J, John J, Pandit S, et al. Musculoskeletal ultrasound imaging of the recently described anterolateral ligament of the knee. Am J Phys Med Rehabil 2014;93:186. [Crossref] [PubMed]
- Oshima T, Nakase J, Numata H, et al. Ultrasonography imaging of the anterolateral ligament using real-time virtual sonography. Knee 2016;23:198-202. [Crossref] [PubMed]
- Capo J, Kaplan DJ, Fralinger DJ, et al. Ultrasonographic visualization and assessment of the anterolateral ligament. Knee Surg Sports Traumatol Arthrosc 2016; [Epub ahead of print]. [Crossref] [PubMed]
- Cavaignac E, Wytrykowski K, Reina N, et al. Ultrasonographic identification of the anterolateral ligament of the knee. Arthroscopy 2016;32:120-6. [Crossref] [PubMed]
- Boeree NR, Watkinson AF, Ackroyd CE, et al. Magnetic resonance imaging of meniscal and cruciate injuries of the knee. J Bone Joint Surg Br 1991;73:452-7. [PubMed]
- Hartigan DE, Carroll KW, Kosarek FJ, et al. Visibility of Anterolateral Ligament Tears in Anterior Cruciate Ligament-Deficient Knees With Standard 1.5-Tesla Magnetic Resonance Imaging. Arthroscopy 2016;32:2061-5. [Crossref] [PubMed]
- Helito CP, Helito PV, Bonadio MB, et al. Correlation of Magnetic Resonance Imaging With Knee Anterolateral Ligament Anatomy: A Cadaveric Study. Orthop J Sports Med 2015;3:2325967115621024 [Crossref] [PubMed]
- Musahl V, Rahnemai-Azar AA, Costello J, et al. The Influence of Meniscal and Anterolateral Capsular Injury on Knee Laxity in Patients With Anterior Cruciate Ligament Injuries. Am J Sports Med 2016;44:3126-31. [Crossref] [PubMed]
- Kittl C, El-Daou H, Athwal KK, et al. The Role of the Anterolateral Structures and the ACL in Controlling Laxity of the Intact and ACL-Deficient Knee. Am J Sports Med 2016;44:345-54. [Crossref] [PubMed]
- Nitri M, Rasmussen MT, Williams BT, et al. An In Vitro Robotic Assessment of the Anterolateral Ligament, Part 2: Anterolateral Ligament Reconstruction Combined With Anterior Cruciate Ligament Reconstruction. Am J Sports Med 2016;44:593-601. [Crossref] [PubMed]
- Parsons EM, Gee AO, Spiekerman C, et al. The biomechanical function of the anterolateral ligament of the knee. Am J Sports Med 2015;43:669-74. [Crossref] [PubMed]
- Rasmussen MT, Nitri M, Williams BT, et al. An In Vitro Robotic Assessment of the Anterolateral Ligament, Part 1: Secondary Role of the Anterolateral Ligament in the Setting of an Anterior Cruciate Ligament Injury. Am J Sports Med 2016;44:585-92. [Crossref] [PubMed]
- Hoshino Y, Araujo P, Ahldén M, et al. Quantitative evaluation of the pivot shift by image analysis using the iPad. Knee Surg Sports Traumatol Arthrosc 2013;21:975-80. [Crossref] [PubMed]
- Katz JW, Fingeroth RJ. The diagnostic accuracy of ruptures of the anterior cruciate ligament comparing the Lachman test, the anterior drawer sign, and the pivot shift test in acute and chronic knee injuries. Am J Sports Med 1986;14:88-91. [Crossref] [PubMed]
- Kaplan N, Wickiewicz TL, Warren RF. Primary surgical treatment of anterior cruciate ligament ruptures. A long-term follow-up study. Am J Sports Med 1990;18:354-8. [Crossref] [PubMed]
- Musahl V, Citak M, O'Loughlin PF, et al. The effect of medial versus lateral meniscectomy on the stability of the anterior cruciate ligament-deficient knee. Am J Sports Med 2010;38:1591-7. [Crossref] [PubMed]
- Musahl V, Ayeni OR, Citak M, et al. The influence of bony morphology on the magnitude of the pivot shift. Knee Surg Sports Traumatol Arthrosc 2010;18:1232-8. [Crossref] [PubMed]
- Fetto JF, Marshall JL. Injury to the anterior cruciate ligament producing the pivot-shift sign. J Bone Joint Surg Am 1979;61:710-4. [Crossref] [PubMed]
- Tanaka M, Vyas D, Moloney G, et al. What does it take to have a high-grade pivot shift? Knee Surg Sports Traumatol Arthrosc 2012;20:737-42. [Crossref] [PubMed]
- Rahnemai-Azar AA, Abebe ES, Johnson P, et al. Increased lateral tibial slope predicts high-grade rotatory knee laxity pre-operatively in ACL reconstruction. Knee Surg Sports Traumatol Arthrosc 2017;25:1170-1176. [Crossref] [PubMed]
- Song GY, Zhang H, Wang QQ, et al. Risk Factors Associated With Grade 3 Pivot Shift After Acute Anterior Cruciate Ligament Injuries. Am J Sports Med 2016;44:362-9. [Crossref] [PubMed]
- Spencer L, Burkhart TA, Tran MN, et al. Biomechanical analysis of simulated clinical testing and reconstruction of the anterolateral ligament of the knee. Am J Sports Med 2015;43:2189-97. [Crossref] [PubMed]
- Sonnery-Cottet B, Lutz C, Daggett M, et al. The Involvement of the Anterolateral Ligament in Rotational Control of the Knee. Am J Sports Med 2016;44:1209-14. [Crossref] [PubMed]
- Saiegh YA, Suero EM, Guenther D, et al. Sectioning the anterolateral ligament did not increase tibiofemoral translation or rotation in an ACL-deficient cadaveric model. Knee Surg Sports Traumatol Arthrosc 2017;25:1086-1092. [Crossref] [PubMed]
- Thein R, Boorman-Padgett J, Stone K, et al. Biomechanical Assessment of the Anterolateral Ligament of the Knee: A Secondary Restraint in Simulated Tests of the Pivot Shift and of Anterior Stability. J Bone Joint Surg Am 2016;98:937-43. [Crossref] [PubMed]
- Sonnery-Cottet B, Thaunat M, Freychet B, et al. Outcome of a Combined Anterior Cruciate Ligament and Anterolateral Ligament Reconstruction Technique With a Minimum 2-Year Follow-up. Am J Sports Med 2015;43:1598-605. [Crossref] [PubMed]
- Schindler OS. Surgery for anterior cruciate ligament deficiency: a historical perspective. Knee Surg Sports Traumatol Arthrosc 2012;20:5-47. [Crossref] [PubMed]
- Warren RF, Marshall JL. Injuries of the Anterior Cruciate and Medial Collateral Ligaments of the Knee: A Long-Term Follow-up of 86 Cases-Part II. Clin Orthop Relat Res 1978;198-211. [PubMed]
- Kennedy JC, Stewart R, Walker DM. Anterolateral rotatory instability of the knee joint. An early analysis of the Ellison procedure. J Bone Joint Surg Am 1978;60:1031-9. [Crossref] [PubMed]
- Strum GM, Fox JM, Ferkel RD, et al. Intraarticular versus intraarticular and extraarticular reconstruction for chronic anterior cruciate ligament instability. Clin Orthop Relat Res 1989;188-98. [PubMed]
- Draganich LF, Reider B, Ling M, et al. An in vitro study of an intraarticular and extraarticular reconstruction in the anterior cruciate ligament deficient knee. Am J Sports Med 1990;18:262-6. [Crossref] [PubMed]
- Engebretsen L, Lew WD, Lewis JL, et al. The effect of an iliotibial tenodesis on intraarticular graft forces and knee joint motion. Am J Sports Med 1990;18:169-76. [Crossref] [PubMed]
- Marcacci M, Zaffagnini S, Giordano G, et al. Anterior Cruciate Ligament Reconstruction Associated With Extra-articular Tenodesis A Prospective Clinical and Radiographic Evaluation With 10-to 13-Year Follow-up. Am J Sports Med 2009;37:707-14. [Crossref] [PubMed]
- Ferretti A, Monaco E, Ponzo A, et al. Combined Intra-articular and Extra-articular Reconstruction in Anterior Cruciate Ligament-Deficient Knee: 25 Years Later. Arthroscopy 2016;32:2039-47. [Crossref] [PubMed]
- Anderson AF, Snyder RB, Lipscomb AB. Anterior cruciate ligament reconstruction a prospective randomized study of three surgical methods. Am J Sports Med 2001;29:272-9. [PubMed]
- Helito CP, Demange MK, Bonadio MB, et al. Radiographic landmarks for locating the femoral origin and tibial insertion of the knee anterolateral ligament. Am J Sports Med 2014;42:2356-62. [Crossref] [PubMed]
Cite this article as: Lucidi GA, Hughes JD, Herbst E, Burnham JM, Musahl V. Role of the anterolateral complex in rotatory instability of the anterior cruciate ligament deficient knee. Ann Joint 2017;2:35.