Effect of anti-inflammatory treatments on patient outcomes and concentrations of inflammatory modulators in the post-surgical and post-traumatic tibiofemoral joint setting: a narrative review
Introduction
Background
Anti-inflammatory drugs are an integral component in the treatment of acute traumatic knee injuries and in post-operative settings. Following acute knee trauma, the synovial fluid within the tibiofemoral joint is in a proinflammatory state, characterized by increased pro-inflammatory cytokines, complement proteins, proteolytic enzymes, and markers of immune system infiltration (1). This proinflammatory state is also seen following surgical procedures. Many of these biomarkers of inflammation persist after the acute stage of trauma or postoperatively and lead to a chronic degenerative change in the synovial environment (2,3). This is most commonly understood as the etiology of post-traumatic osteoarthritis (PTOA) (4). Further, it has been proposed that anti-inflammatory treatments administered in the acute post-traumatic or post-surgical setting may be beneficial in delaying or preventing the onset of PTOA.
Rationale and knowledge gap
There is a paucity of literature concerning biomarker analysis and treatment modality in human studies. This is most likely attributed to the risk of infection from aspiration in post-traumatic and post-surgical settings, as well as difficulty in study recruitment within these settings. Because of this, many studies in this field have been done using animal models. Despite this, the modulation of biomarkers in animal models can serve as a basis of understanding in humans. The effects of different anti-inflammatory therapeutics in clinical outcomes are more well defined, and comparisons can be made among different modalities.
Objective
The purpose of this review is three-fold: to accurately summarize the effects of anti-inflammatory treatments in post-traumatic and post-surgical tibiofemoral joint settings, assess clinical outcomes associated with different treatment modalities, and identify areas of potential research concerning biomarker analysis and treatment modulation in human studies. We present this article in accordance with the Narrative Review reporting checklist (available at https://aoj.amegroups.com/article/view/10.21037/aoj-23-55/rc).
Methods
This review provides an overview of the literature surrounding anti-inflammatory treatments. The principal information sources have been drawn from a literature search of MEDLINE and the Cochrane Database of Systematic Reviews, as well as cross-referencing among studies found in these searches. An analysis of these searches included clinical trials, animal studies, observational studies, and laboratory studies. Studies were included based on analysis of inflammatory biomarkers and clinical outcomes. Relevant studies concerning mechanisms of action were also consulted. Single case reports were not included. There was no exclusion criteria based on date of publication. A summary of the methods can be seen in Table 1.
Table 1
Items | Specification |
---|---|
Date of search (specified to date, month and year) | 5/10/2023 |
Databases and other sources searched | MEDLINE, Cochrane Database of Systematic Reviews |
Search terms used | MEDLINE: “knee” OR tibiofemoral joint: AND “anti-inflammatory”; Cochrane Database of Systematic Reviews: “anti-inflammatory” AND “knee” |
Timeframe | January 1970–May 2023 |
Inclusion and exclusion criteria | Full text peer reviewed articles clinical trials, animal studies, observational studies, and laboratory studies in English were included for review; single case studies were excluded |
Selection process | Titles and abstracts were independently reviewed by two authors (C.P.O. and M.I.K.), consensus obtained by reviewing full text |
Anti-inflammatory effects on inflammatory modulators and clinical outcomes
Non-steroidal anti-inflammatory drugs (NSAIDs)
NSAIDs are the most conservative method of treating inflammation in acute knee trauma settings. In addition to being analgesics, they also provide anti-inflammatory benefits through the modulation and inhibition of the enzyme cyclooxygenase (COX) (5). There are two COX isoenzymes, COX-1 and COX-2, and both have different enzymatic effects (6). COX-1 is constitutively expressed and COX-2 is inducibly expressed during inflammatory responses. The majority of NSAIDs are nonselective COX inhibitors and inhibit both isoenzymes. The reduction in eicosanoid synthesis mitigates the inflammatory response, leading to a downregulation of additional biomarkers of inflammation. The inflammatory response and modulation by NSAIDs can be seen in Figure 1.
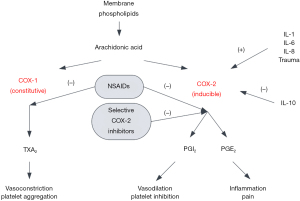
NSAID treatment induces a dose-dependent reduction in cytokines related to inflammation in the knee with a reduction in synovial fluid biomarkers of interleukin (IL)-6, tissue necrosis factor alpha (TNF-α), and vascular endothelial growth factor (VEGF) (7). The reduction in IL-6 can be partially attributed to the inhibition of prostaglandin E2 (PGE2) synthesis, which in turn inhibits IL-6 expression and production by bone cells. Reduced IL-6 levels are also caused by the reduction in TNF-α expression. NSAIDs have been shown to inactivate MAPKs in knee articular cartilage, with a subsequent reduction in TNF-α and other pro-inflammatory cytokines (8). Gallelli et al. demonstrated that this reduction is not restricted to articular cartilage and also reduces mitogen-activated protein kinase (MAPK) activity in the synovial membrane (7). They proposed that the inhibition of TNF-α with NSAID treatment led to decreased expression and a reduction in chronic inflammation. VEGF downregulation is also induced by NSAID treatment and reduces the inflammation brought on by the increase in vascularity at the site of injury. However, this effect can also be deleterious to healing, and can be considered an adverse effect in the context of prolonged NSAID use, as it may lead to worse healing outcomes (9).
The downregulation of MAPK also reduces the synthesis of matrix metalloproteinases (MMPs), which are primarily associated with extracellular matrix degradation and cytokine activation. NSAIDS have been shown to significantly inhibit MMP-1 and MMP-3 production (10). This is in conflict with the stimulatory effects of NSAIDs on IL-1β production which induces the expression of several MMPs (10). Increased PGE2 levels also suppress the induction of MMPs by IL-1β, indicating that NSAID administration should increase these proteinases. However, it is possible that this downregulation is through a different pathway, with induction of transforming growth factor-beta one (TGF-β1) being proposed as a likely factor given its inhibitory effects on IL-1β MMP production (11). The levels of other MMPs have been shown to be relatively unaffected by NSAID administration (10,12). The reduction of MMP concentration may be one of the causative factors in the inhibition of initial degeneration in the acute setting of trauma and the prevention of further degenerative changes within the tibiofemoral joint. However, additional studies are needed to elucidate the inhibitory effects of NSAIDs on these protein levels.
Clinically, the effects of NSAID treatment in acute knee trauma and post-surgery settings is more defined, but there is still a gap in the literature concerning human studies. One of the most important findings is that perioperative NSAID administration does not affect soft tissue healing (13,14). This is because the healing process of soft tissue within the knee relies on pathways that are not inhibited by NSAIDs (13). However, this is not the case in bone and tendon-to-bone healing. Prostaglandins are required for osteoblast and osteoclast activation, and the inhibition of COX-2 by NSAIDs and selective COX-2 inhibitors block this necessary inflammatory pathway (15). This deleterious effect on tendon-to-bone healing has been shown to be increased with respect to selective COX-2 inhibitors (15). In one study focusing on rotator cuff repair, celecoxib was associated with significantly higher retear rates compared to ibuprofen or tramadol within a 24-month follow-up period (16). Despite this, Ge et al. demonstrated that celecoxib did not lead to worse outcomes or failure rates compared to tramadol in patients undergoing arthroscopic anterior cruciate ligament (ACL) reconstruction at 12 months follow-up (17).
Overall, NSAIDs have been demonstrated to be beneficial in the acute setting not only for their analgesic effects, but in their modulation of degenerative biomarkers. NSAIDs can be safely administered with minimal side effects, and their early inhibitory effects can provide a protective role in the degeneration seen in knee trauma. Prolonged NSAID use may be deleterious to healing, as the inhibition of the pro-inflammatory state brought on by COX-2 has been shown to prolong the healing process (18). However, the acute effects of NSAIDs make them a useful treatment for acute knee injury and post-surgical settings.
Steroids
Dexamethasone
Dexamethasone is a glucocorticoid that has demonstrated anti-inflammatory and chondroprotective effects. It is a wide-spectrum steroid that has also been shown to improve proteoglycan synthesis and decrease glycosaminoglycans (GAG) loss in injured cartilage (19,20). While it can be given orally, intra-articular injections allow for greater concentrations at the tibiofemoral joint compared to systemic administration.
In an animal model of trauma, a single injection (0.5 mg/kg) administered immediately after osteochondral drill injury led to significantly decreased IL-1β, IL-6, IL-8, and MMP-1, -3, and -13 at 2 months (21). In the same model, repeated injection every three days for three weeks after injury led to significantly decreased IL-1β and type I collagen expression and decreased MMP-3 levels. In an in vitro study with human chondrocytes, Dexamethasone injection has also been shown to inhibit nitric oxide (NO), COX-2, IL-1, and IL-6 production with IL-17 stimulation, indicating a blunting of IL-17 activated MAP kinases (22). In short, dexamethasone has demonstrated the ability to rescue cartilage matrix loss and convey chondrocyte viability by inhibition of these pro-inflammatory, degenerative biomarkers.
As a single therapeutic, intra-articular dexamethasone has a half-life of 4 hours (23). Because of this, different formulations of drug have been developed aimed at prolonging concentration levels and increased penetration at the site of injection. Dexamethasone has been shown to have prolonged anti-inflammatory effects when linked with avidin, a small cationic molecule (24). This was tested in an animal model, when compared to a single bolus of dexamethasone, avidin-dexamethasone led to a prolonged decrease in inflammation of up to 50% for three weeks after injury, with full cartilage penetration of avidin confirmed by immunostaining observed as well (19). IL-1β, MMP-1, and ADAMTS-5 gene expression reduction has been demonstrated upon administration of avidin-dexamethasone as well, but did not result in decreased MMP-3 and -13 levels or prevent GAG loss. A second formulation of dexamethasone with avidin is utilizing avidin-biotinylated polyethylene glycol (PEG), which allowed for an initial burst provided by a single dexamethasone shot and the prolonged release and penetration of the avidin formulation in degraded bovine cartilage (24,25). Compared to a single dose of dexamethasone, which inhibited GAG loss for eight days, this formulation prevented GAG loss for three weeks. Another formulation in clinical trials is a liposomal encapsulation of dexamethasone injected intraarticularly, which also prolongs the release of the drug (26). While biomarkers were not analyzed in this study, pain was reduced for 24 weeks after a single dose, with no reported adverse effects.
Clinically, the use of dexamethasone can be considered controversial because while it may result in short term reduction in pain and inflammation it has also been shown that repeated doses exert catabolic effects on healthy cartilage and reduce cell proliferation (27). Because of this, low-dose prolonged release formulations may be able to maintain the anabolic dosage without the initial catabolic effects that occur at the onset of administration related to higher concentrations. As single dose prolonged release formulations become available, this risk may be mitigated; however, these formulations are currently not clinically used. At low doses, it has been found that dexamethasone can stimulate human tendon stem cells, but high doses resulted in decreased cell proliferation (28). It also results in non-tenocyte differentiation at high doses, resulting in non-tendinous tissues increasing the risk of subsequent rupture.
The dosing and frequency of dexamethasone administration must be weighed in the treatment of traumatic knee settings. While it has been shown to have anti-inflammatory and anti-degenerative effects when administered in low doses, higher single doses and repeated doses have been shown to increase articular damage (27,29). Further studies are needed to determine the effective dose for acute trauma settings. A summary of reviewed studies is highlighted in Table 2.
Table 2
Author | Model | Dosing | Findings |
---|---|---|---|
Heard et al. (21) | Rabbit model, osteochondral drill injury | 0.5 mg/kg single dose intra-articular injection | Decreased IL-1β, IL-6, IL-8, and MMP-1, -3, and -13 at 2 months |
0.5 mg/kg intra-articular injection every three days for three weeks | Decreased IL-1β and type I collagen expression and decreased MMP-3 levels at 2 months | ||
Shalom-Barak et al. (22) | In-vitro isolated human chondrocytes, stimulated with IL-17 | 100 nM | Decreased NO, COX-2, IL-1, and IL-6 production |
Bajpayee et al. (19) | Rabbit model, ACL transection | 100 μM single dose intra-articular injection of avidin-dexamethasone | 50% reduction in inflammation at 3 weeks |
Decreased IL-1β, MMP-1, and ADAMTS-5 expression | |||
No change in GAG loss at three weeks | |||
He et al. (25) | In-vitro cytokine challenged bovine cartilage models | 4:1 Dex-PEG: avidin at 0.010 mmol concentration | Prevention of GAG loss at three weeks |
Hunter et al. (26) | Phase II clinical trial | 12 or 18 mg single intra-articular injection of liposomal encapsulated dexamethasone | Improved pain at 24 weeks |
IL, interleukin; NO, nitric oxide; COX, cyclooxygenase; ACL, anterior cruciate ligament; MMP, matrix metalloproteinase; Dex-PEG, dexamethasone-polyethylene glycol; GAG, glycosaminoglycan.
Triamcinolone acetonide (TCA)
In acute traumatic knee injuries, the administration of TCA, another clinically approved glucocorticosteroid, carries many of the same pros and cons as dexamethasone. It has been shown to reduce cartilage degradation when administered within two weeks of ACL injury (30). It was also observed that CTX-II (C-terminal cross-linking telopeptide of type II collagen) levels, which is associated with collagen type II breakdown, were significantly reduced in patients receiving TCA (30). There was no difference at 5 weeks in levels of inflammatory markers such as TSG-6 (tumor necrosis factor stimulated gene-6), IL1-α, IL-1β, or IL-1ra between patients treated with or without TCA, with an increase in these markers over that period. Patients reported improved pain outcomes, but not at a significantly greater level than those in the placebo group.
In a porcine model, a single TCA injection administered immediately after ACL transection resulted in significantly reduced levels of C1 and C2 collagen fragments in synovial fluid, mononuclear leukocytes, and decreased CX3CR1 chemokine receptor expression after 14 days compared to the group not receiving a TCA injection (31). In the group treated with TCA, there were also significantly increased levels of MMP-8 at 14 days (31). In both of these studies, MMP-1 and -2 levels were not significantly reduced, potentially indicating a lack of IL-1β modulation (30,31).
TCA also a fast rate of clearance from the joint, resulting in the need for sustained-release formulations (32). This has resulted in polyester amide (PEA) and poly lactic-co-glycolic acid (PLGA) microsphere systems being developed (33,34). In animal models, PEA microspheres were detected after 8 weeks from the initial treatment (33). PEA microsphere delivery has also been shown to be superior to PLGA delivery in reducing pain symptoms (33). However, while this resulted in prolonged decreased inflammation, there was no effect on preventing cartilage damage.
Clinically, there is one PLGA microsphere formulation that is currently approved (35). It has been shown to maintain residence in the human knee joint for 12 weeks and reduced pain in 74% of patients (36,37). It was also observed that there was no impact on cartilage radiographically after two doses with the second dose administered at a median time of 16.6 weeks (37). However, repeated use of traditional isolated TCA over the course of two years has been shown to decrease cartilage volume (38).
These findings support that TCA administration in acute trauma settings may have chondroprotective effects but limited effects on controlling inflammation. Long term administration of TCA is not recommended and has been observed to cause articular cartilage damage, but may be beneficial in acute settings (38). A summary of findings in reviewed studies can be seen in Table 3.
Table 3
Author | Model | Dosage | Findings |
---|---|---|---|
Latterman et al. (30) | Randomized clinical trial of patients experiencing ACL injury | 40 mg TCA intra-articular injection at 4 days and 2 weeks after injury or placebo | Reduced cartilage degeneration. CTX-II levels decreased. No difference in pain outcomes |
No difference in TSG-6, IL1-α, IL-1β, or IL-1ra at five weeks | |||
Sieker et al. (31) | Porcine model, ACL transection | 20 mg TCA single dose intra-articular injection immediately after ACL transection | Decreased C1, C2 collagen fragments |
Decreased CX3CR1, MMP-8 expression | |||
No difference in MMP-1 and -2 | |||
Rudnik-Jansen et al. (33) | Osteoarthritis induced murine model | 5 µL of PEA microspheres loaded with triamcinolone (50 mg/mL particles) single dose intra-articular | Reduced pain symptoms compared to non-PEA microsphere injection. Microsphere presence detected at 8 weeks. No prevention in cartilage damage |
Spitzer et al. (37) | Clinical trial (phase 3b) in patients with symptomatic knee osteoarthritis | 5 mL TCA-PLGA microsphere formulation administered at day 1 and a second injection at week 12, 16, 20, or 24 | Reduced pain, no impact on cartilage degeneration at 17 weeks |
McAlindon et al. (38) | Randomized clinical trial | 40 mg TCA every 3 months versus normal saline | Significant loss of cartilage at 2 years. No difference in pain at two years |
TCA, triamcinolone acetonide; ACL, anterior cruciate ligament; CTX-II, C-terminal cross-linking telopeptide of type II collagen; MMP, matrix metalloproteinase; PEA, polyester amide; PLGA, poly lactic-co-glycolic acid.
Biologics
Hyaluronic acid (HA)
HA is a GAG that is endogenously produced by type B synoviocytes and fibroblasts (39). HA provides synovial fluid viscosity, as well as providing lubrication, shock absorption, and elasticity within the tibiofemoral joint (1). In inflammatory conditions and mechanical trauma settings, the concentration of HA is decreased as well as being fragmented. This is due to infiltrative macrophages that internalize HA and degrade it (40). In pro-inflammatory states, there is also an upregulation in hyaluronidases within the synovial fluid, resulting in further cleavage (41). This also presents a problem in examining the effects of HA, as differing levels of these hyaluronidases in different settings can affect the efficacy of exogenous HA injections.
HA injections as viscosupplementation have been shown to acutely increase joint lubrication (42). In addition to this lubricative effect, high molecular weight (HMW) HA supplementation has also been shown to have anti-inflammatory effects. In an animal model, long chain hyaluronate had a dose dependent decrease in phagocytosis by macrophages, while short chain hyaluronate did not change the level of phagocytosis (43). HA injections have also demonstrated alterations in leukocyte function related to migration, chemotaxis, adherence, and proliferation (39,44). These effects are believed to be related to prostaglandin inhibition and cAMP stimulation (45). In one human osteoarthritis study, MMP-9 concentrations were decreased in HA injections, compared to no change in a group treated with corticosteroid injection at 5 weeks (46). In another study evaluating inflammation markers after tibial plateau fractures, HMW HA administration resulted in decreased IL-1β and TNF-α and increased levels of IL-10 and tissue inhibitor of metalloproteinases 1 (TIMP-1) (47). HA has also been shown to not have any effect on IL-6 levels when administered in acute injury settings (48). While the viscoelastic benefits of HA are well understood, further study is necessary to develop an understanding of anti-inflammatory efficacy.
Clinically, the literature on HA is heterogenous due to differences in formulation and dosing protocols in human studies. HA injections following ACL reconstruction provided no significant benefit in postoperative pain scores, swelling, or functional outcomes compared to controls (49-51). There is also a paucity of literature concerning HA in human acute trauma settings, but animal model studies have been done that demonstrate mixed effects (52). Notably, it was found that intraarticular injections of HA had a profound impact on healing and protection in articular cartilage after meniscal injuries, but insignificant protection after ACL injury in agreement with previously mentioned studies (53,54). It was also demonstrated that it had little to no effect on healing of articular cartilage.
While human studies are limited, and have demonstrated limited efficacy in post-surgical settings, animal studies have demonstrated that HA may have limited use as a protective agent in soft tissue injuries in traumatic settings. Additional human studies are necessary to further elucidate the role that HA may have in both biomarkers of inflammation and clinical outcomes. Reviewed studies can be seen in Table 4.
Table 4
Author | Model | Dosage | Findings |
---|---|---|---|
Forrester et al. (43) | In-vitro peritoneal murine macrophages | HMW HA (4.6×105 Da) | High molecular weight HA caused a dose-dependent inhibition of phagocytosis, while low molecular weight HA did not |
LMW HA (9.0×104 Da) | |||
Shimizu et al. (46) | Clinical trial in patients with osteoarthritis | 25 mg HA intra-articular injection single dose versus corticosteroid | Decreased MMP-9 levels compared to corticosteroid group at 5 weeks |
Huang et al. (47) | Human fibroblast synoviocytes harvested during tibial plateau fracture open reduction and internal fixation | HMW HA (6.0×106 Da) | Decreased IL-1β and TNF-α, increased IL-10 and TIMP-1 |
LMW HA (5.0×105 Da) |
HMW, high molecular weight; HA, hyaluronic acid; MMP, matrix metalloproteinase; LMW, low molecular weight; IL, interleukin; TNF-α, tissue necrosis factor alpha; TIMP-1, tissue inhibitor of metalloproteinases 1.
Platelet-rich plasma (PRP)
PRP is a solution of highly concentrated platelets and growth factors in plasma that can be injected into the intra-articular space. PRP injections have been increasingly used as a treatment of chronic injuries and degenerative joint diseases. Despite their increase in frequency in clinical settings, PRP injections lack standardization with variability in composition, delivery technique, and administration location in each commercial preparation. PRP can also be administered alone, or in combination with HA or other anti-inflammatories (55,56). The short biological half-life of the components within PRP also highlights that frequency of injections plays a role in potential therapeutic effects, with no agreement on what this frequency is (57). This variability, in conjunction with a poorly understood mechanism of action, makes the use of PRP in the context of traumatic knee injuries controversial.
A discussion on PRP effects on inflammatory biomarkers is also limited by the heterogeneity in PRP systems, with no consensus on the use of leukocyte rich PRP, leukocyte-poor PRP, or pure PRP (58). In one study, there was no significant difference in IL-1B or TNF-a levels in patients treated with PRP (low-leukocyte autologous conditioned plasma system) with a downward trend of these markers at 24 weeks following injection (59). It is possible that this downregulation was also due to native healing and progressive down regulation of proinflammatory cytokines. Another leukocyte-poor PRP study demonstrated that PRP does not inhibit the inflammatory response by chondrocytes stimulated with TNF-α (60). COX-2, prostaglandin E synthase (PTGES), and IL-17 levels, were expectedly upregulated by TNF-α exposure and were not affected by the addition of PRP. This is in contrast with other studies that observed an inhibitory effect in inflammation in cartilaginous tissue (61-63). All three of these studies used different formulations of PRP, again highlighting the heterogeneity in PRP utilization.
PRP formulations have been studied in multiple surgical settings. PRP administration in conjunction with meniscus repair has been shown to have some efficacy, with earlier clinical and radiographic evidence of healing being observed, as well as long-term improvement in function (64,65). This is believed to be due to the anti-inflammatory effects of PRP that promote meniscal healing. ACL reconstruction augmented with PRP has also been shown to be beneficial, in both subjective and clinical outcomes (66). PRP has been shown to improve ACL graft maturation compared to controls (66). In an MRI study, 100% of patients returned to baseline MRI signal intensity comparted to 78% in the control group at six months, indicating a faster healing process (67). This is further confirmed by another study which showed a return to baseline signal intensity of 177 days in patients treated with PRP compared to 369 days in controls (68). Despite these earlier signs of healing, there was no difference in clinical outcomes at 2 years between groups in both studies (67,68). Concerning high tibial osteotomies (HTO), HTO combined with PRP is associated with improved clinical outcomes after 6 months (69,70). However, one study found that PRP mixed into allograft bone was associated with non-union compared to allograft alone throughout a 4-year follow-up period (71). In the context of total knee arthroplasty, PRP therapy following the surgery was associated with short term improvements, but did not have any overall improved function (72).
While it is possible that PRP may prove to have distinct, modulatory effects in the future, the specific effects of PRP on inflammatory modulators remains to be clearly defined. There is also limited literature surrounding the effect of PRP on outcomes in trauma settings. However, the literature supports the use of PRP in certain surgical contexts, most notably meniscus repair. The findings of PRP formulations on biomarker modulation can be seen in Table 5.
Table 5
Author | Model | Dosage | Findings |
---|---|---|---|
Cole et al. (59) | Randomized clinical trial (phase II) in patients with osteoarthritis | 5 mL low leukocyte autologous conditioned plasma single dose intra-articular injection | No significant decrease in IL-1B or TNF-a levels compared to patients receiving LMW HA |
Rikkers et al. (60) | In vitro human osteoarthritic chondrocytes | Leukocyte poor PRP | No inhibition of inflammatory response when stimulated with TNF-α |
Mishra et al. (61) | In vitro human mesenchymal stem cells | Leukocyte poor, inactivated PRP | PRP enhanced stem cell proliferation and chondrogenic differentiation |
Pereira et al. (62) | In vitro human chondrocytes | PRP derivative (platelet lysate) | PRP resulted in repression of COX-2 and a reduction in inflammation |
Bendinelli et al. (63) | In vitro human chondrocytes | Activated PRP | Decreased inflammation as a result of HGF |
IL, interleukin; TNF-α, tissue necrosis factor alpha; LMW, low molecular weight; HA, hyaluronic acid; PRP, platelet-rich plasma; COX, cyclooxygenase; HGF, hepatocyte growth factor.
IL-1 receptor antagonist (IL-1ra)
The use of IL-1ra has become a topic of interest in preventing the inflammatory progression seen in knee trauma. The hypothesized mechanism of action is that irreversible inhibition of IL-1 receptors (IL-1ra) leads to a decrease in IL-1β inflammation and degenerative processes (73,74). Exogenous IL-1ra levels typically increase during the first 2 weeks post-trauma, but eventually become undetectable (4). This is in contrast with IL-1β which remains significantly elevated up to 1.5 months after trauma. Considering the different timelines of expression, IL-1ra therapeutics may be able to delay this change in differential expression and limit the effect of IL-1β and the induction of degenerative changes.
Clinical outcomes have been shown to improve with IL-1ra (anakinra) intra-articular injections in the short term, but this effect was lost after one month, indicating that a single injection had no influence on decreasing knee pain or function (75). In another study, IL-1ra injection 2 weeks post ACL injury resulted in a significant decrease in synovial IL-1α and serum HA, but there was no effect on IL-1β levels, indicating that IL-1ra may only delay the effects brought on by IL-1β (76). Continuous systemic IL-1ra doses have also been observed to increase IL-6 levels in a murine model, leading to more articular damage (77).
Due to the observed detrimental effects of daily IL-1ra administration, and a short half-life of 4 hours, formulations focused on sustained release of have been tested (78,79). In a murine model, the use of cross-linked human elastin-like polypeptides (ELP) resulted in detectable levels of IL-1ra for 5 days (78). This formulation also resulted in a moderate but short effect in a decrease in MMP-3 levels for 4 weeks post-injury. A PLGA formulation of IL-1ra has been observed to prevent synovial inflammation and cartilage degeneration at 4 weeks post-injection (79).
While IL-1ra injections may be promising, additional human studies are needed to determine their effectiveness in acute settings for improvement in outcomes. Biomarker findings from the reviewed studies are summarized in Table 6.
Table 6
Author | Model | Dosage | Findings |
---|---|---|---|
Chevalier et al. (75) | Randomize clinical trial in patients with osteo | 50 or 15 mg single dose intra-articular anakinra | No significant difference in knee pain or function |
Kraus et al. (76) | Patients with acute ACL tear | 150 mg single dose intra-articular anakinra 2 weeks post injury | Decreased knee pain and improved function over two weeks follow up Decreased synovial IL-1α and serum HA, no change in IL-1β |
Furman et al. (77) | Murine model subjected to closed articular fracture of knee | 0.9 mg single dose intra-articular injection immediately after injury or continuous systemic infusion (1.0 mg per day) | Significant reduction in cartilage degeneration in intra-articular injection. Increased degeneration in continuous systemic infusion |
Kimmerling et al. (78) | Murine model subjected to closed articular fracture of knee | 0.45 mg IL-1ra: ELP formulation single dose intra-articular injection | Detection of IL-1ra for 5 days. Moderate decrease in MMP-3 |
Elsaid et al. (79) | Murine mode with ACL transection | 5 mg/mL PLGA: IL-1ra single dose intraarticular | Decreased inflammation compared to non PLGA conjugated IL-1ra formulation |
IL-1ra, IL-1 receptor antagonist; ACL, anterior cruciate ligament; IL, interleukin; HA, hyaluronic acid; ELP, elastin-like polypeptides; MMP, matrix metalloproteinase; PLGA, poly lactic-co-glycolic acid.
Anti-IL-6 receptor antibody
Given the role of IL-6 as a causal cytokine of acute inflammation, the potential inhibition of it has been considered in the treatment of knee trauma as well as the prevention of PTOA. Tocilizumab and sarilumab are two monoclonal antibodies that competitively inhibit membrane bound and secreted IL-6 receptors, and are commonly used to treat rheumatoid arthritis (80,81). No clinical studies have assessed IL-6 inhibitors as a treatment to prevent PTOA or reduce inflammation in a post-surgical setting.
However, the inhibition of IL-6 has been shown to prevent apoptosis in rat chondrocytes (82). It has also been shown to prevent the production of IL-6 from subchondral bone, as well as decreasing cartilage lesions and subchondral bone sclerosis in murine models of ACL injuries (83). While the literature is limited on the efficacy of anti-IL-6 receptor antibodies in acute settings, future research may show the beneficial effects of administration in the prevention of further degeneration.
Natural anti-inflammatories
There are a variety of natural anti-inflammatory substances that may be beneficial in the prevention of degenerative processes. These compounds include ginseng, turmeric, omega-3 fatty acids, green tea, and arnica, as well as many others. A brief overview of these compounds and their mechanisms of action can be seen in Figure 2.
The active components of ginseng are ginsenosides, a diverse category of compounds, which have been shown to downregulate pro-inflammatory cytokines such as TNF-α, IL-1β, and IL-6 as well as the downregulation of COX-2 (84). Ginsenosides have also been implicated in the polarization of M2 macrophages and the resolution of inflammation (85). Ginsenoside Ro specifically has been shown to suppress IL-1β induced apoptosis of rat chondrocytes (86). Further, it downregulated the expression of MMP-3 and -9, demonstrating both anti-apoptotic and anti-inflammatory effects. Ginseng also has limited side effects and can be used as a supplement to reduce inflammation in acute settings. However, there are no clinical studies assessing ginseng as a single therapeutic in acute settings, and additional research is needed in human subjects to assess the efficacy of this substance.
Turmeric is another alternative substance that has demonstrated anti-inflammatory effects. Curcumin is the component that has demonstrated the greatest anti-inflammatory effect, although other components have been shown to possess anti-inflammatory properties (87,88). The anti-inflammatory effect of curcumin is also considered to be dose and time independent (89). The literature on biomarker modulation is heterogenous with one meta-analysis of 5,870 patients showing a significant decrease in IL-6 and TNF-α levels (89), while another meta-analysis of 1,344 patients demonstrating that turmeric resulted in no difference in IL-1, IL-6, of TNF levels compared to controls (90). To date, there are no studies assessing the use of turmeric in either post-traumatic or post-surgical settings. Despite this, turmeric has been shown to beneficial in some cases in reducing inflammation and may be considered as a supplemental treatment in conjunction with more traditional approaches.
Omega-3 fatty acids, commonly derived from marine sources, are one of the two major families of polyunsaturated fatty acids, with the other being omega-6 (91). Both of these fatty acids are eventually incorporated into cellular membranes, and the differential composition of these membranes have been shown to relate to the potential for inflammatory responses (92). Omega-6 has been shown to have a pro-inflammatory profile primarily associated with its conversion to arachidonic acid (ARA) and the subsequent synthesis of eicosanoids. In contrast with this pro-inflammatory profile, omega-3 demonstrates anti-inflammatory characteristics. These anti-inflammatory characteristics are due to two modulatory effects. The first is that omega-3 is converted into eicosatetraenoic acid (EPA) and docosahexaenoic acid (DHA) which results in decreased availability of ARA (92). The second effect is that EPA derived eicosanoids are less biologically potent compared to ARA-derived eicosanoids, resulting in decreased inflammation (93). EPA has also been shown to inhibit ARA and decreased COX-2 gene expression (93). This effect has also been shown to be dose dependent (94). Omega-3 fatty acids have also been observed to be inflammation resolving, with the products of EPA and DHA preventing neutrophil infiltration and inhibition of TNF-α and IL-1β production (95). As with other natural anti-inflammatory supplements, omega-3 fatty acids can be considered beneficial in reducing inflammation, but have not been studied extensively in acute knee settings.
The active compound in green tea, epigallocaetechin-3-gallate, has been shown to have anti-inflammatory effects through multiple pathways (96). In animal models, green tea has been shown to repress gene and protein expression of TNF-α and IL-1β, and IL-6 (97). Further, MMP-2 and -9 levels have been shown to be decreased as well with the oral administration of epigallocaetechin-3-gallate (98). When used in conjunction with NSAIDs, green tea has been shown to result in better functional knee scores in patients with osteoarthritis compared to NSAIDs alone (99). Epigallocaetechin-3-gallate has not been evaluated as a standalone treatment in acute settings, but may well serve as an adjunctive therapeutic when paired with other treatment modalities.
Arnica is another plant-based complementary therapy that is widely used as an anti-inflammatory. It has many different formulations, including topical and oral routes of administration. Arnica has also been shown to work in a dose dependent manner (100). While there are countless arnica extracts derived from the Asteraceae (daisy) family, Arnica montana L. is the most commonly used extract (101). The most active component of Arnica is helenalin, although other derivatives also have similar mechanisms of action (102). Helenalin has been shown in vitro to inhibit nuclear factor kappa B (NF-κB) and a subsequent downregulation of IL-1, -2, -6, -8, and TNF- α in human lymphocytes and epithelial cells (103). It has also been shown to inhibit neutrophil migration and chemotaxis as well as downregulation of and 5-lipooxygenase and its subsequent leukotrienes in vitro (104,105). COX-2 has also been shown to be downregulated, due to NF-κB inhibition, in a murine model (106). Unlike many other natural remedies, Arnica has been studied in an acute knee setting. Brinkhaus et al. (107) tested oral administrations Arnica as a supplement in conjunction with standard post-operative care and found that it significantly reduced swelling and pain after cruciate ligament reconstruction, but not in arthroscopy, artificial knee joint implantation. However, conventional pain medications were also available upon request in this trial, and not controlled for, which may complicate the findings. Despite this, Arnica has been shown to biochemically inhibit pro-inflammatory markers and may be beneficial as a supplemental treatment.
Discussion
This review covers the different types of anti-inflammatory interventions that can be used in post-traumatic and post-surgical settings. The most important finding of this review is that there is no single therapeutic that is clearly superior to other modalities, and that each option has different indications. At this time, there are also limited studies that evaluate anti-inflammatory interventions in human trauma models, causing a reliance on animal models to determine biomarker profiles. Furthermore, anti-inflammatories are often used as a means of analgesia and are often not considered based on their risk profile in long term settings. While many of these interventions have risks concerning healing and degeneration with prolonged, repeated use, they are still all relatively effective in acute settings when indicated. Additionally, as prolonged release formulations of different interventions become available, many of these risks associated with repeated use can be mitigated.
Referring to the interventions themselves, NSAIDs, dexamethasone, and TCA are the most well studied and have been shown to all be beneficial in acute settings. This is especially true in the context of soft tissue injuries. Both HA and PRP have been shown to beneficial in reducing inflammation, but they both lack the standardization necessary to determine their efficacy. IL-1ra and IL-6 antibodies show promise, but more study is needed before these can be considered as a treatment option. Finally, the natural anti-inflammatory compounds mentioned in this study are not exhaustive but cover some of the most commonly used adjunctive treatments for inflammation. They can all be safely used with minimal side effects, but none of them have demonstrated the ability to be used as an isolated treatment in the context of trauma or surgery.
While studies have begun to elucidate the biomarker modulation profile of each of these therapeutics, it is still clear that additional research needs to be done. Post surgical models are more well defined, and post-traumatic settings need to be more thoroughly explored. While surgery may mimic the pro-inflammatory profile that is brought on by trauma, it is obvious that this is more limited in damage, making direct comparisons difficult.
This research is subject to some limitations. Firstly, this review was not carried out systematically, meaning it is possible that additional sources were not identified and analyzed. Secondly, there is a lack of literature surrounding trauma models and anti-inflammatory therapeutics, making it difficult to draw direct conclusions in these settings. There is also heterogeneity in the reported variables of each study in this review, in both outcomes and measurements. This makes it difficult to compare different modalities to each other. Future studies should aim to be comparative in nature to determine if there are superior outcomes clinically and from a biomarker standpoint.
Conclusions
There are a variety of anti-inflammatory therapeutic options that can be used in post-traumatic and post-surgical knee settings that have demonstrated efficacy in improving clinical outcomes. Despite these improvements, there is still a considerable lack in the literature concerning biomarkers of inflammation in these settings in conjunction with anti-inflammatory treatments. While many conventional therapeutics are indicated in the acute setting, it is important to weigh the deleterious effect of prolonged anti-inflammatory treatment on the progression of healing in the context of knee injuries.
Acknowledgments
Funding: None.
Footnote
Provenance and Peer Review: This article was commissioned by the editorial office, Annals of Joint for the series “Inflammation of the Tibiofemoral Joint: Inflammatory Mediators, Treatment, and Long-Term Effects”. The article has undergone external peer review.
Reporting Checklist: The authors have completed the Narrative Review reporting checklist. Available at https://aoj.amegroups.com/article/view/10.21037/aoj-23-55/rc
Peer Review File: Available at https://aoj.amegroups.com/article/view/10.21037/aoj-23-55/prf
Conflicts of Interest: All authors have completed the ICMJE uniform disclosure form (available at https://aoj.amegroups.com/article/view/10.21037/aoj-23-55/coif). The series “Inflammation of the Tibiofemoral Joint: Inflammatory Mediators, Treatment, and Long-Term Effects” was commissioned by the editorial office without any funding or sponsorship. N.N.D. served as the unpaid Guest Editor of the series and serves as an unpaid editorial board member of Annals of Joint from August 2022 to July 2024. R.F.L. is a consultant for Ossur, Smith & Nephew, and Responsive Arthroscopy; collects royalties from Ossur, Smith & Nephew, Elsevier, and Arthrex; has research grants from Ossur, Smith & Nephew, AANA, AOSSM; is on the committees for ISAKOS, AOSSM, AANA; and is on the editorial boards for AJSM, JEO, KSSTA, JKS, JISPT, OTSM. The authors have no other conflicts of interest to declare.
Ethical Statement: The authors are accountable for all aspects of the work in ensuring that questions related to the accuracy or integrity of any part of the work are appropriately investigated and resolved.
Open Access Statement: This is an Open Access article distributed in accordance with the Creative Commons Attribution-NonCommercial-NoDerivs 4.0 International License (CC BY-NC-ND 4.0), which permits the non-commercial replication and distribution of the article with the strict proviso that no changes or edits are made and the original work is properly cited (including links to both the formal publication through the relevant DOI and the license). See: https://creativecommons.org/licenses/by-nc-nd/4.0/.
References
- Khella CM, Horvath JM, Asgarian R, et al. Anti-Inflammatory Therapeutic Approaches to Prevent or Delay Post-Traumatic Osteoarthritis (PTOA) of the Knee Joint with a Focus on Sustained Delivery Approaches. Int J Mol Sci 2021;22:8005. [Crossref] [PubMed]
- Schenker ML, Mauck RL, Ahn J, et al. Pathogenesis and prevention of posttraumatic osteoarthritis after intra-articular fracture. J Am Acad Orthop Surg 2014;22:20-8. [Crossref] [PubMed]
- Lohmander LS, Englund PM, Dahl LL, et al. The long-term consequence of anterior cruciate ligament and meniscus injuries: osteoarthritis. Am J Sports Med 2007;35:1756-69. [Crossref] [PubMed]
- Khella CM, Asgarian R, Horvath JM, et al. An Evidence-Based Systematic Review of Human Knee Post-Traumatic Osteoarthritis (PTOA): Timeline of Clinical Presentation and Disease Markers, Comparison of Knee Joint PTOA Models and Early Disease Implications. Int J Mol Sci 2021;22:1996. [Crossref] [PubMed]
- Vane JR, Botting RM. Anti-inflammatory drugs and their mechanism of action. Inflamm Res 1998;47:S78-87. [Crossref] [PubMed]
- Vane JR. Inhibition of prostaglandin synthesis as a mechanism of action for aspirin-like drugs. Nat New Biol 1971;231:232-5. [Crossref] [PubMed]
- Gallelli L, Galasso O, Falcone D, et al. The effects of nonsteroidal anti-inflammatory drugs on clinical outcomes, synovial fluid cytokine concentration and signal transduction pathways in knee osteoarthritis. A randomized open label trial. Osteoarthritis Cartilage 2013;21:1400-8. [Crossref] [PubMed]
- Tsutsumi R, Ito H, Hiramitsu T, et al. Celecoxib inhibits production of MMP and NO via down-regulation of NF-kappaB and JNK in a PGE2 independent manner in human articular chondrocytes. Rheumatol Int 2008;28:727-36. [Crossref] [PubMed]
- Manzano-Moreno FJ, Costela-Ruiz VJ, Melguizo-Rodríguez L, et al. Inhibition of VEGF gene expression in osteoblast cells by different NSAIDs. Arch Oral Biol 2018;92:75-8. [Crossref] [PubMed]
- Syggelos SA, Giannopoulou E, Gouvousis PA, et al. In vitro effects of non-steroidal anti-inflammatory drugs on cytokine, prostanoid and matrix metalloproteinase production by interface membranes from loose hip or knee endoprostheses. Osteoarthritis Cartilage 2007;15:531-42. [Crossref] [PubMed]
- Yuan W, Varga J. Transforming growth factor-beta repression of matrix metalloproteinase-1 in dermal fibroblasts involves Smad3. J Biol Chem 2001;276:38502-10. [Crossref] [PubMed]
- Efstathiou M, Settas L. The effect of non-steroidal anti-inflammatory drugs on matrix metalloproteinases levels in patients with osteoarthritis. Mediterr J Rheumatol 2017;28:133-41. [Crossref] [PubMed]
- Constantinescu DS, Campbell MP, Moatshe G, et al. Effects of Perioperative Nonsteroidal Anti-inflammatory Drug Administration on Soft Tissue Healing: A Systematic Review of Clinical Outcomes After Sports Medicine Orthopaedic Surgery Procedures. Orthop J Sports Med 2019;7:2325967119838873. [Crossref] [PubMed]
- Chen MR, Dragoo JL. The effect of nonsteroidal anti-inflammatory drugs on tissue healing. Knee Surg Sports Traumatol Arthrosc 2013;21:540-9. [Crossref] [PubMed]
- Sauerschnig M, Stolberg-Stolberg J, Schmidt C, et al. Effect of COX-2 inhibition on tendon-to-bone healing and PGE2 concentration after anterior cruciate ligament reconstruction. Eur J Med Res 2018;23:1. [Crossref] [PubMed]
- Oh JH, Seo HJ, Lee YH, et al. Do Selective COX-2 Inhibitors Affect Pain Control and Healing After Arthroscopic Rotator Cuff Repair? A Preliminary Study. Am J Sports Med 2018;46:679-86. [Crossref] [PubMed]
- Ge H, Liu C, Shrestha A, et al. Do Nonsteroidal Anti-Inflammatory Drugs Affect Tissue Healing After Arthroscopic Anterior Cruciate Ligament Reconstruction? Med Sci Monit 2018;24:6038-43. [Crossref] [PubMed]
- Pountos I, Georgouli T, Calori GM, et al. Do nonsteroidal anti-inflammatory drugs affect bone healing? A critical analysis. ScientificWorldJournal 2012;2012:606404. [Crossref] [PubMed]
- Bajpayee AG, De la Vega RE, Scheu M, et al. Sustained intra-cartilage delivery of low dose dexamethasone using a cationic carrier for treatment of post traumatic osteoarthritis. Eur Cell Mater 2017;34:341-64. [Crossref] [PubMed]
- Lu YC, Evans CH, Grodzinsky AJ. Effects of short-term glucocorticoid treatment on changes in cartilage matrix degradation and chondrocyte gene expression induced by mechanical injury and inflammatory cytokines. Arthritis Res Ther 2011;13:R142. [Crossref] [PubMed]
- Heard BJ, Barton KI, Chung M, et al. Single intra-articular dexamethasone injection immediately post-surgery in a rabbit model mitigates early inflammatory responses and post-traumatic osteoarthritis-like alterations. J Orthop Res 2015;33:1826-34. [Crossref] [PubMed]
- Shalom-Barak T, Quach J, Lotz M. Interleukin-17-induced gene expression in articular chondrocytes is associated with activation of mitogen-activated protein kinases and NF-kappaB. J Biol Chem 1998;273:27467-73. [Crossref] [PubMed]
- Johnson DB, Lopez MJ, Kelley B. Dexamethasone. In: StatPearls [Internet]. Treasure Island (FL): StatPearls Publishing; 2023.
- Bajpayee AG, Scheu M, Grodzinsky AJ, et al. Electrostatic interactions enable rapid penetration, enhanced uptake and retention of intra-articular injected avidin in rat knee joints. J Orthop Res 2014;32:1044-51. [Crossref] [PubMed]
- He T, Zhang C, Vedadghavami A, et al. Multi-arm Avidin nano-construct for intra-cartilage delivery of small molecule drugs. J Control Release 2020;318:109-23. [Crossref] [PubMed]
- Hunter D, Chang CC, Wei JC, et al. Single intra-articular injection of TLC599 provided sustained pain relief through 24 weeks in participants with symptomatic knee osteoarthritis. Osteoarthritis and Cartilage 2019;27:S87-8. [Crossref]
- Black R, Grodzinsky AJ. Dexamethasone: chondroprotective corticosteroid or catabolic killer? Eur Cell Mater 2019;38:246-63. [Crossref] [PubMed]
- Zhang J, Keenan C, Wang JH. The effects of dexamethasone on human patellar tendon stem cells: implications for dexamethasone treatment of tendon injury. J Orthop Res 2013;31:105-10. [Crossref] [PubMed]
- Zeng C, Lane NE, Hunter DJ, et al. Intra-articular corticosteroids and the risk of knee osteoarthritis progression: results from the Osteoarthritis Initiative. Osteoarthritis Cartilage 2019;27:855-62. [Crossref] [PubMed]
- Lattermann C, Jacobs CA, Proffitt Bunnell M, et al. A Multicenter Study of Early Anti-inflammatory Treatment in Patients With Acute Anterior Cruciate Ligament Tear. Am J Sports Med 2017;45:325-33. [Crossref] [PubMed]
- Sieker JT, Ayturk UM, Proffen BL, et al. Immediate Administration of Intraarticular Triamcinolone Acetonide After Joint Injury Modulates Molecular Outcomes Associated With Early Synovitis. Arthritis Rheumatol 2016;68:1637-47. [Crossref] [PubMed]
- Larsen C, Ostergaard J, Larsen SW, et al. Intra-articular depot formulation principles: role in the management of postoperative pain and arthritic disorders. J Pharm Sci 2008;97:4622-54. [Crossref] [PubMed]
- Rudnik-Jansen I, Colen S, Berard J, et al. Prolonged inhibition of inflammation in osteoarthritis by triamcinolone acetonide released from a polyester amide microsphere platform. J Control Release 2017;253:64-72. [Crossref] [PubMed]
- Kumar A, Bendele AM, Blanks RC, et al. Sustained efficacy of a single intra-articular dose of FX006 in a rat model of repeated localized knee arthritis. Osteoarthritis Cartilage 2015;23:151-60. [Crossref] [PubMed]
- Paik J, Duggan ST, Keam SJ. Triamcinolone Acetonide Extended-Release: A Review in Osteoarthritis Pain of the Knee. Drugs 2019;79:455-62. [Crossref] [PubMed]
- Kraus VB, Conaghan PG, Aazami HA, et al. Synovial and systemic pharmacokinetics (PK) of triamcinolone acetonide (TA) following intra-articular (IA) injection of an extended-release microsphere-based formulation (FX006) or standard crystalline suspension in patients with knee osteoarthritis (OA). Osteoarthritis Cartilage 2018;26:34-42. [Crossref] [PubMed]
- Spitzer AI, Richmond JC, Kraus VB, et al. Safety and Efficacy of Repeat Administration of Triamcinolone Acetonide Extended-release in Osteoarthritis of the Knee: A Phase 3b, Open-label Study. Rheumatol Ther 2019;6:109-24. [Crossref] [PubMed]
- McAlindon TE, LaValley MP, Harvey WF, et al. Effect of Intra-articular Triamcinolone vs Saline on Knee Cartilage Volume and Pain in Patients With Knee Osteoarthritis: A Randomized Clinical Trial. JAMA 2017;317:1967-75. [Crossref] [PubMed]
- Brockmeier SF, Shaffer BS. Viscosupplementation therapy for osteoarthritis. Sports Med Arthrosc Rev 2006;14:155-62. [Crossref] [PubMed]
- Culty M, Nguyen HA, Underhill CB. The hyaluronan receptor (CD44) participates in the uptake and degradation of hyaluronan. J Cell Biol 1992;116:1055-62. [Crossref] [PubMed]
- Moreland LW. Intra-articular hyaluronan (hyaluronic acid) and hylans for the treatment of osteoarthritis: mechanisms of action. Arthritis Res Ther 2003;5:54-67. [Crossref] [PubMed]
- Balazs EA, Denlinger JL. Viscosupplementation: a new concept in the treatment of osteoarthritis. J Rheumatol Suppl 1993;39:3-9. [PubMed]
- Forrester JV, Balazs EA. Inhibition of phagocytosis by high molecular weight hyaluronate. Immunology 1980;40:435-46. [PubMed]
- Ghosh P. The role of hyaluronic acid (hyaluronan) in health and disease: interactions with cells, cartilage and components of synovial fluid. Clin Exp Rheumatol 1994;12:75-82. [PubMed]
- Punzi L, Schiavon F, Cavasin F, et al. The influence of intra-articular hyaluronic acid on PGE2 and cAMP of synovial fluid. Clin Exp Rheumatol 1989;7:247-50. [PubMed]
- Shimizu M, Higuchi H, Takagishi K, et al. Clinical and biochemical characteristics after intra-articular injection for the treatment of osteoarthritis of the knee: prospective randomized study of sodium hyaluronate and corticosteroid. J Orthop Sci 2010;15:51-6. [Crossref] [PubMed]
- Huang TL, Hsu HC, Yang KC, et al. Effect of different molecular weight hyaluronans on osteoarthritis-related protein production in fibroblast-like synoviocytes from patients with tibia plateau fracture. J Trauma 2010;68:146-52. [Crossref] [PubMed]
- Roth A, Mollenhauer J, Wagner A, et al. Intra-articular injections of high-molecular-weight hyaluronic acid have biphasic effects on joint inflammation and destruction in rat antigen-induced arthritis. Arthritis Res Ther 2005;7:R677-86. [Crossref] [PubMed]
- Huang MH, Yang RC, Chou PH. Preliminary effects of hyaluronic acid on early rehabilitation of patients with isolated anterior cruciate ligament reconstruction. Clin J Sport Med 2007;17:242-50. [Crossref] [PubMed]
- Chau JY, Chan WL, Woo SB, et al. Hyaluronic acid instillation following arthroscopic anterior cruciate ligament reconstruction: a double-blinded, randomised controlled study. J Orthop Surg (Hong Kong) 2012;20:162-5. [Crossref] [PubMed]
- Di Martino A, Tentoni F, Di Matteo B, et al. Early Viscosupplementation After Anterior Cruciate Ligament Reconstruction: A Randomized Controlled Trial. Am J Sports Med 2016;44:2572-8. [Crossref] [PubMed]
- Edouard P, Rannou F, Coudeyre E. Animal evidence for hyaluronic acid efficacy in knee trauma injuries. Review of animal-model studies. Phys Ther Sport 2013;14:116-23. [Crossref] [PubMed]
- Kobayashi K, Amiel M, Harwood FL, et al. The long-term effects of hyaluronan during development of osteoarthritis following partial meniscectomy in a rabbit model. Osteoarthritis Cartilage 2000;8:359-65. [Crossref] [PubMed]
- Mihara M, Higo S, Uchiyama Y, et al. Different effects of high molecular weight sodium hyaluronate and NSAID on the progression of the cartilage degeneration in rabbit OA model. Osteoarthritis Cartilage 2007;15:543-9. [Crossref] [PubMed]
- Mariani E, Canella V, Cattini L, et al. Leukocyte-Rich Platelet-Rich Plasma Injections Do Not Up-Modulate Intra-Articular Pro-Inflammatory Cytokines in the Osteoarthritic Knee. PLoS One 2016;11:e0156137. [Crossref] [PubMed]
- Terada S, Ota S, Kobayashi M, et al. Use of an antifibrotic agent improves the effect of platelet-rich plasma on muscle healing after injury. J Bone Joint Surg Am 2013;95:980-8. [Crossref] [PubMed]
- Szwedowski D, Szczepanek J, Paczesny Ł, et al. The Effect of Platelet-Rich Plasma on the Intra-Articular Microenvironment in Knee Osteoarthritis. Int J Mol Sci 2021;22:5492. [Crossref] [PubMed]
- Lana JF, Huber SC, Purita J, et al. Leukocyte-rich PRP versus leukocyte-poor PRP - The role of monocyte/macrophage function in the healing cascade. J Clin Orthop Trauma 2019;10:S7-S12. [Crossref] [PubMed]
- Cole BJ, Karas V, Hussey K, et al. Hyaluronic Acid Versus Platelet-Rich Plasma: A Prospective, Double-Blind Randomized Controlled Trial Comparing Clinical Outcomes and Effects on Intra-articular Biology for the Treatment of Knee Osteoarthritis. Am J Sports Med 2017;45:339-46. [Crossref] [PubMed]
- Rikkers M, Dijkstra K, Terhaard BF, et al. Platelet-Rich Plasma Does Not Inhibit Inflammation or Promote Regeneration in Human Osteoarthritic Chondrocytes In Vitro Despite Increased Proliferation. Cartilage 2021;13:991S-1003S. [Crossref] [PubMed]
- Mishra A, Tummala P, King A, et al. Buffered platelet-rich plasma enhances mesenchymal stem cell proliferation and chondrogenic differentiation. Tissue Eng Part C Methods 2009;15:431-5. [Crossref] [PubMed]
- Pereira RC, Scaranari M, Benelli R, et al. Dual effect of platelet lysate on human articular cartilage: a maintenance of chondrogenic potential and a transient proinflammatory activity followed by an inflammation resolution. Tissue Eng Part A 2013;19:1476-88. [Crossref] [PubMed]
- Bendinelli P, Matteucci E, Dogliotti G, et al. Molecular basis of anti-inflammatory action of platelet-rich plasma on human chondrocytes: mechanisms of NF-κB inhibition via HGF. J Cell Physiol 2010;225:757-66. [Crossref] [PubMed]
- Kaminski R, Kulinski K, Kozar-Kaminska K, et al. A Prospective, Randomized, Double-Blind, Parallel-Group, Placebo-Controlled Study Evaluating Meniscal Healing, Clinical Outcomes, and Safety in Patients Undergoing Meniscal Repair of Unstable, Complete Vertical Meniscal Tears (Bucket Handle) Augmented with Platelet-Rich Plasma. Biomed Res Int 2018;2018:9315815. [Crossref] [PubMed]
- Pujol N, Salle De Chou E, Boisrenoult P, et al. Platelet-rich plasma for open meniscal repair in young patients: any benefit? Knee Surg Sports Traumatol Arthrosc 2015;23:51-8. [Crossref] [PubMed]
- Vavken P, Sadoghi P, Murray MM. The effect of platelet concentrates on graft maturation and graft-bone interface healing in anterior cruciate ligament reconstruction in human patients: a systematic review of controlled trials. Arthroscopy 2011;27:1573-83. [Crossref] [PubMed]
- Orrego M, Larrain C, Rosales J, et al. Effects of platelet concentrate and a bone plug on the healing of hamstring tendons in a bone tunnel. Arthroscopy 2008;24:1373-80. [Crossref] [PubMed]
- Radice F, Yánez R, Gutiérrez V, et al. Comparison of magnetic resonance imaging findings in anterior cruciate ligament grafts with and without autologous platelet-derived growth factors. Arthroscopy 2010;26:50-7. [Crossref] [PubMed]
- Dong C, Zhao C, Wang F. Clinical benefit of high tibial osteotomy combined with the intervention of platelet-rich plasma for severe knee osteoarthritis. J Orthop Surg Res 2022;17:405. [Crossref] [PubMed]
- Zhang Q, Xu W, Wu K, et al. Intra-articular Pure Platelet-Rich Plasma Combined With Open-Wedge High Tibial Osteotomy Improves Clinical Outcomes and Minimal Joint Space Width Compared With High Tibial Osteotomy Alone in Knee Osteoarthritis: A Prospective Study. Arthroscopy 2022;38:476-85. [Crossref] [PubMed]
- Giuseffi SA, Replogle WH, Shelton WR. Opening-Wedge High Tibial Osteotomy: Review of 100 Consecutive Cases. Arthroscopy 2015;31:2128-37. [Crossref] [PubMed]
- Muchedzi TA, Roberts SB. A systematic review of the effects of platelet rich plasma on outcomes for patients with knee osteoarthritis and following total knee arthroplasty. Surgeon 2018;16:250-8. [Crossref] [PubMed]
- Jacques C, Gosset M, Berenbaum F, et al. The role of IL-1 and IL-1Ra in joint inflammation and cartilage degradation. Vitam Horm 2006;74:371-403. [Crossref] [PubMed]
- Fields JK, Günther S, Sundberg EJ. Structural Basis of IL-1 Family Cytokine Signaling. Front Immunol 2019;10:1412. [Crossref] [PubMed]
- Chevalier X, Goupille P, Beaulieu AD, et al. Intraarticular injection of anakinra in osteoarthritis of the knee: a multicenter, randomized, double-blind, placebo-controlled study. Arthritis Rheum 2009;61:344-52. [Crossref] [PubMed]
- Kraus VB, Birmingham J, Stabler TV, et al. Effects of intraarticular IL1-Ra for acute anterior cruciate ligament knee injury: a randomized controlled pilot trial (NCT00332254). Osteoarthritis Cartilage 2012;20:271-8. [Crossref] [PubMed]
- Furman BD, Mangiapani DS, Zeitler E, et al. Targeting pro-inflammatory cytokines following joint injury: acute intra-articular inhibition of interleukin-1 following knee injury prevents post-traumatic arthritis. Arthritis Res Ther 2014;16:R134. [Crossref] [PubMed]
- Kimmerling KA, Furman BD, Mangiapani DS, et al. Sustained intra-articular delivery of IL-1RA from a thermally-responsive elastin-like polypeptide as a therapy for post-traumatic arthritis. Eur Cell Mater 2015;29:124-39; discussion 139-40. [Crossref] [PubMed]
- Elsaid KA, Ubhe A, Shaman Z, et al. Intra-articular interleukin-1 receptor antagonist (IL1-ra) microspheres for posttraumatic osteoarthritis: in vitro biological activity and in vivo disease modifying effect. J Exp Orthop 2016;3:18. [Crossref] [PubMed]
- Yip RML, Yim CW. Role of Interleukin 6 Inhibitors in the Management of Rheumatoid Arthritis. J Clin Rheumatol 2021;27:e516-24. [Crossref] [PubMed]
- Singh JA, Furst DE, Bharat A, et al. 2012 update of the 2008 American College of Rheumatology recommendations for the use of disease-modifying antirheumatic drugs and biologic agents in the treatment of rheumatoid arthritis. Arthritis Care Res (Hoboken) 2012;64:625-39. [Crossref] [PubMed]
- Zhou R, Wu X, Wang Z, et al. Interleukin-6 enhances acid-induced apoptosis via upregulating acid-sensing ion channel 1a expression and function in rat articular chondrocytes. Int Immunopharmacol 2015;29:748-60. [Crossref] [PubMed]
- Wu X, Cao L, Li F, et al. Interleukin-6 from subchondral bone mesenchymal stem cells contributes to the pathological phenotypes of experimental osteoarthritis. Am J Transl Res 2018;10:1143-54. [PubMed]
- Kim JH, Yi YS, Kim MY, et al. Role of ginsenosides, the main active components of Panax ginseng, in inflammatory responses and diseases. J Ginseng Res 2017;41:435-43. [Crossref] [PubMed]
- Kang S, Park SJ, Lee AY, et al. Ginsenoside Rg(3) promotes inflammation resolution through M2 macrophage polarization. J Ginseng Res 2018;42:68-74. [Crossref] [PubMed]
- Zhang XH, Xu XX, Xu T. Ginsenoside Ro suppresses interleukin-1β-induced apoptosis and inflammation in rat chondrocytes by inhibiting NF-κB. Chin J Nat Med 2015;13:283-9. [Crossref] [PubMed]
- Prasad S, Gupta SC, Tyagi AK, et al. Curcumin, a component of golden spice: from bedside to bench and back. Biotechnol Adv 2014;32:1053-64. [Crossref] [PubMed]
- Aggarwal BB, Yuan W, Li S, et al. Curcumin-free turmeric exhibits anti-inflammatory and anticancer activities: Identification of novel components of turmeric. Mol Nutr Food Res 2013;57:1529-42. [Crossref] [PubMed]
- Naghsh N, Musazadeh V, Nikpayam O, et al. Profiling Inflammatory Biomarkers following Curcumin Supplementation: An Umbrella Meta-Analysis of Randomized Clinical Trials. Evid Based Complement Alternat Med 2023;2023:4875636. [Crossref] [PubMed]
- White CM, Pasupuleti V, Roman YM, et al. Oral turmeric/curcumin effects on inflammatory markers in chronic inflammatory diseases: A systematic review and meta-analysis of randomized controlled trials. Pharmacol Res 2019;146:104280. [Crossref] [PubMed]
- Innes JK, Calder PC. Omega-6 fatty acids and inflammation. Prostaglandins Leukot Essent Fatty Acids 2018;132:41-8. [Crossref] [PubMed]
- Calder PC. Omega-3 fatty acids and inflammatory processes: from molecules to man. Biochem Soc Trans 2017;45:1105-15. [Crossref] [PubMed]
- Calder PC. Marine omega-3 fatty acids and inflammatory processes: Effects, mechanisms and clinical relevance. Biochim Biophys Acta 2015;1851:469-84. [Crossref] [PubMed]
- Rees D, Miles EA, Banerjee T, et al. Dose-related effects of eicosapentaenoic acid on innate immune function in healthy humans: a comparison of young and older men. Am J Clin Nutr 2006;83:331-42. [Crossref] [PubMed]
- Serhan CN, Chiang N, Van Dyke TE. Resolving inflammation: dual anti-inflammatory and pro-resolution lipid mediators. Nat Rev Immunol 2008;8:349-61. [Crossref] [PubMed]
- Ohishi T, Goto S, Monira P, et al. Anti-inflammatory Action of Green Tea. Antiinflamm Antiallergy Agents Med Chem 2016;15:74-90. [Crossref] [PubMed]
- Marinovic MP, Morandi AC, Otton R. Green tea catechins alone or in combination alter functional parameters of human neutrophils via suppressing the activation of TLR-4/NFκB p65 signal pathway. Toxicol In Vitro 2015;29:1766-78. [Crossref] [PubMed]
- Demeule M, Brossard M, Pagé M, et al. Matrix metalloproteinase inhibition by green tea catechins. Biochim Biophys Acta 2000;1478:51-60. [Crossref] [PubMed]
- Hashempur MH, Sadrneshin S, Mosavat SH, et al. Green tea (Camellia sinensis) for patients with knee osteoarthritis: A randomized open-label active-controlled clinical trial. Clin Nutr 2018;37:85-90. [Crossref] [PubMed]
- Berges C, Fuchs D, Opelz G, et al. Helenalin suppresses essential immune functions of activated CD4+ T cells by multiple mechanisms. Mol Immunol 2009;46:2892-901. [Crossref] [PubMed]
- Iannitti T, Morales-Medina JC, Bellavite P, et al. Effectiveness and Safety of Arnica montana in Post-Surgical Setting, Pain and Inflammation. Am J Ther 2016;23:e184-97. [Crossref] [PubMed]
- Alvarez-Hernández E, César Casasola-Vargas J, Lino-Pérez L, et al. Complementary and alternative medicine in patients attending a rheumatology department for the first time. Analysis of 800 patients. Reumatol Clin 2006;2:183-9. [PubMed]
- Lyss G, Schmidt TJ, Merfort I, et al. Helenalin, an anti-inflammatory sesquiterpene lactone from Arnica, selectively inhibits transcription factor NF-kappaB. Biol Chem 1997;378:951-61. [Crossref] [PubMed]
- Hall IH, Starnes CO Jr, Lee KH, et al. Mode of action of sesquiterpene lactones as anti-inflammatory agents. J Pharm Sci 1980;69:537-43. [Crossref] [PubMed]
- Tornhamre S, Schmidt TJ, Näsman-Glaser B, et al. Inhibitory effects of helenalin and related compounds on 5-lipoxygenase and leukotriene C(4) synthase in human blood cells. Biochem Pharmacol 2001;62:903-11. [Crossref] [PubMed]
- Verma N, Tripathi SK, Sahu D, et al. Evaluation of inhibitory activities of plant extracts on production of LPS-stimulated pro-inflammatory mediators in J774 murine macrophages. Mol Cell Biochem 2010;336:127-35. [Crossref] [PubMed]
- Brinkhaus B, Wilkens JM, Lüdtke R, et al. Homeopathic arnica therapy in patients receiving knee surgery: results of three randomised double-blind trials. Complement Ther Med 2006;14:237-46. [Crossref] [PubMed]
Cite this article as: Olson CP, Kennedy MI, DePhillipo NN, Tagliero AJ, LaPrade RF, Kennedy NI. Effect of anti-inflammatory treatments on patient outcomes and concentrations of inflammatory modulators in the post-surgical and post-traumatic tibiofemoral joint setting: a narrative review. Ann Joint 2024;9:9.