Principles for optimizing anterior cruciate ligament reconstruction outcomes in elite athletes: a review of current techniques
Introduction
Anterior cruciate ligament (ACL) tears are one of the most common sport-related injuries, occurring in over 200,000 patients per year in the United States. Greater than 3% of athletes sustain an ACL tear within a 4-year window of sport participation and the incidence of ACL tears continues to rise for all levels of competition, a trend that includes elite athletes (1-5). Females are at increased risk for ACL injury and women’s gymnastics, soccer and basketball represent three of the four highest-risk sports, behind American football (3,6). Furthermore, ACL injuries in elite athletes have been found to occur at increased rates in competition versus practice. There is a reported 20-fold increase in the ACL match play injury rate in European professional soccer compared to practice, with similar findings being reported in National Collegiate Athletic Association (NCAA) Football and the National Football League (NFL) (7-9).
The frequency of dynamic, “high risk” movements in sports affects the risk of ACL injury in elite level athletes. A study by Kobayashi et al. reviewed the mechanisms of ACL injury in 1,700 patients and reported that noncontact injury was the most common mechanism for ACL tears. The authors also reported dynamic alignment in valgus and external tibial rotation to be a significant risk factor at the time of injury, resulting in significant strain to the ACL during the loading phase of knee flexion (9).
Patient outcomes for all-inside ACL reconstruction (ACLR) techniques are generally quite favorable, but have been observed to vary based on sport type. A retrospective study by Mai et al. found that ACLR in NFL athletes leads to a worse prognosis compared to other professional sports, with decreased on-field performance and shorter overall career length. In comparison, ACLR in NHL athletes was associated with the most favorable outcomes based on the same criteria. The physical demands imposed on the ACL graft during competition may explain these differences. Running and cutting in American football subject the surgically reconstructed knee to increased forces and could result in increased knee laxity, graft strain and return to sport (RTS) time compared with other sports which are less reliant upon these movements (10). This demonstrates the need not only for adherence to the full length of ACLR RTS protocol, but also the potential desirability of tailoring RTS protocol to individual sport demands.
The treatment of ACL rupture in elite level athletes poses a unique challenge because the patient must be able to return to sport at the same level of performance. Marginal postoperative changes in clinical or functional outcomes, which would not substantively affect return to activity in the general population, may result in decreased statistical performance and shorter careers. Return to “near-optimal” performance may not be sufficient. The current literature on ACLR outcomes in elite athletes is mixed. A recent systematic review found that 83% of professional athletes returned to a similar level of sport following ACLR. However, other studies have found decreased statistical performance and fewer starts for professional soccer players with ACLR (4,11,12). The variability in outcomes may be explained by the range in treatment options, graft selection, ACLR techniques, individualized anatomy of the patient, and the method and rigor of post-operative rehabilitation programs. The aim of this article is to summarize the existing techniques and principles used to achieve optimal results for ACLR in elite level athletes.
Graft selection for elite athletes
The ideal graft for ACLR in elite athletes should reproduce the anatomical and biomechanical properties of the native ACL, establish robust fixation, and facilitate rapid biologic integration (1). Beyond graft choice, promising innovations in orthobiologics have aimed to accelerate the ligamentization and osseointegration of grafts using techniques such as autologous tissue-engineered polyethylene terephthalate, but more research is needed in human models (13). Numerous studies have evaluated allogenic, synthetic, and autogenous grafts based on these guiding principles. However, despite the preponderance of literature on the subject, no current graft fulfills these criteria to the extent of the native ACL, and there remains no gold standard for anatomical ACL graft choice (14,15). Therefore, it is the surgeon’s responsibility to evaluate the advantages and disadvantages for each graft and make an optimal selection based upon the patient’s sport, individualized anatomy, and history of previous ACLR (1,12).
Bone-patellar tendon-bone (BTB) autograft
BTB autograft (Figure 1) is widely considered the premium graft choice for ACLR in elite athletes, 68% of Major League Soccer (MLS) team orthopedic surgeons prefer single-bundle BTB for ACLR and similar trends have been reported for the surgical treatment of NFL, NHL and US Olympic Ski and Snowboard athletes (16-19). Many surgeons prefer a BTB autograft due to its significant stiffness and tensile strength, which has been reported to exceed that of the native ACL (20). Past studies have reported decreased anterior knee laxity following BTB autograft compared to other autografts (20-22). The BTB autograft is also favored because the graft results in more rapid and durable bone integration compared to soft tissue grafts, decreasing the risk of future ACL revision (1,12). A study by Gifstad et al. found that BTB autografts resulted in significantly decreased risk of revision compared to ACLR with hamstring (HS) autografts in 45,998 patients with primary ACLR (23).
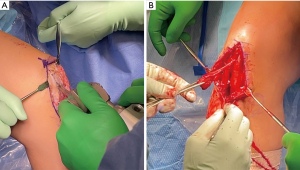
Despite these advantages, BTB autografts have been linked to increased complication rates when compared to other graft types (1). A majority of complications occur during graft harvesting and can result in patellar tendon rupture, patellar tendonitis, and patellar fractures (24). In addition, BTB autograft has an increased risk of post-operative anterior knee pain that has been reported to occur in 5% to 55% of BTB ACLR (1,21). Therefore, the BTB autograft should be considered with care in sports such as wrestling in which the athlete is required to kneel, and in sports which require force across the anterior aspect of the knee for extended periods.
HS autograft
The HS autograft (Figure 2) offers several characteristics that make it advantageous for specific ACLR populations. Compared to BTB autograft, the HS autograft has a wider mid-substance surface area and lower overall rates of complications such as anterior knee pain, loss of knee extension strength, and range of motion (25,26). In addition, ACLR with HS autografts can be used for single-bundle or double-bundle ACLRs and has been associated with reliable clinical outcomes: studies have reported that 69% of patients return to pre-surgical levels following the HS autograft while 8% of patients experience residual knee laxity greater than 5 mm on KT-1000 testing (1). HS autografts are also the preferred choice for ACLR in skeletally immature athletes due to the decreased risk of local physis bone arrest, compared to BTB autograft (27).
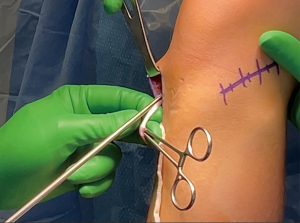
Despite these advantages, HS autografts have been associated with increased healing and bone-graft integration times due to the absence of stabilizing bone plugs. Increased rates of infection with HS autografts have also been reported (12). HS ACLR may also result in decreased knee flexion torque in the post-operative period (1), and thus may be an inferior choice for elite athletes who rely heavily on HS flexion such as sprinters, wrestlers, gymnasts, and long-distance runners. However, additional literature has suggested this effect generally resolves one year after surgery and HS strength recovery can be accelerated with biceps femoris training (28). Overall, the HS autograft remains an effective graft option for anatomic ACLR in elite athletes. When performed, the surgeon may consider a concomitant lateral extraarticular tenodesis (LET) which has been reported to result in decreased rates of graft failure when compared with isolated HS autograft ACLR (29), though there is speculation that this effect may be caused by the forced increase in recovery time resulting from concurrent LET that allows further maturation of the graft before it is significantly stressed.
Quadriceps tendon (QT) autograft
The QT autograft (Figure 3) has emerged as a potential graft option in recent years because it can be harvested with or without a bone block and as a full or partial thickness graft (30). The QT autograft has increased length, tensile strength and mid-substance cross-sectional area compared to the BTB autograft and therefore may be advantageous in elite athletes with a broad ACL footprint (12). In a study of 144 patients with primary ACLR, Han et al. reported comparable clinical outcomes for QT and BTB autografts (31). Notably, the authors found a significantly decreased incidence of post-operative anterior knee pain in the QT group (31). QT autograft also minimizes the risk of injuring the saphenous nerve, which remains a frequent complication of BTB graft harvesting (1). Furthermore, QT autograft has not been reported to have the increased risk of infection that accompanies HS autograft (32).
Comparing differences in knee stability, patient satisfaction and self-reported functional outcomes, a recent systematic review by Slone et al. found no significant differences when comparing QT versus HS and BTB autografts (33). Drawbacks to QT autografts include the risk of patellar fracture during graft harvesting, significant bleeding due to the profuse vascular supply of the QT, and rectus femoris retraction (12,33,34). The use of QT autograft in elite athletes remains largely uncharted due to the lack of trials with long-term follow up and the hesitancy surrounding potential loss of quadriceps function beyond that already associated with disuse during recovery. Additional high quality studies resulting in favorable clinical outcomes may encourage more surgeons to select the QT autograft for ACLR in elite athletes.
Allograft
The use of allografts for high-level athletes remains controversial. While the lack of donor site morbidity may be attractive in high-level athletes trying to return to play as efficiently as possible, the associated cost is higher reported rates of failure. Because of the reported increase in allograft rupture, especially in young patients, we do not recommend allografts as the first line choice for anatomic ACLR in elite athletes (12,35). A systematic review by Wasserstein et al. found a significantly increased pooled failure rate of 25% in ACLR with allograft compared to 9.6% with autograft (36). Additional evidence suggests that allograft usage in ACLR results in delayed return to sport versus autograft, potentially because autograft is more easily incorporated into host bone (37). In rare cases, allograft ACLR may be justified for elite athletes with a multiligament knee injury or significant compromise of autograft tissue, but autograft remains the preference of most surgeons (38).
Graft preparation and fixation
Graft preparation, tensioning, and fixation are essential considerations for successful ACLR, particularly in high-level athletes (see Table 1, surgical pearls and pitfalls). Failure to properly account for graft properties may result in significant postoperative knee laxity due to graft elongation and heightens the risk of ACLR failure. Debate exists in the literature regarding the proper graft pretensioning and preconditioning techniques that reproduce the biomechanics of the native ACL in various graft choices. This section will review principles behind pre- and peri-operative graft considerations for ACLR.
Table 1
Techniques | Pearls | Pitfalls |
---|---|---|
ACLR (all techniques) | Patient positioning to allow flexion up to 120º with stable varus/valgus maneuvering may be achieved by using a leg holder placed proximally and angled cephalad to slightly raise the knee | Failure to properly position patient prior to procedure may lead to inadequate visualization and/or angles for tunnels |
An accessory medial portal should be created prior to the femoral tunnel creation | Careful attention should be paid to not disrupt the meniscal root attachments during tunnel placement | |
Preservation of some ACL tissue ‘stumps’ helps guide appropriate tunnel placements | Improper tunnel placement (femoral tunnel too vertical, too posterior; tibial tunnel too medial, lateral or posterior) will result in a non-anatomic and/or compromised reconstruction with higher risk of graft failure | |
After graft positioning but before tensioning, cycling the knee may help remove slack and avoid impingement | Lack of physician coordination and involvement with physical therapy may result in improper timing of return to sport and increased levels of either failure or stiffness | |
Use of dynamic force ACL brace in pre- and post-operative settings reduces strain on the injured or newly reconstructed ACL | ||
BTB autograft | The senior author’s preferred sizing is 10 mm tendon width, with 20 mm × 10 mm × 10 mm patellar and 25 mm × 10 mm × 10 mm tibial bone block | Failure to create a sufficient incision may lead to accidental marking and harvesting of insufficiently sized bone blocks |
Arthroscopy portals may be created through the harvest site incision | Failure to create adequate cuts with the oscillating saw may result in fracture when retrieving the blocks via osteotome | |
Care should be taken to dissect patellar paratenon from both sides of the graft | Failure to properly plan/mark the bone cuts may result in too large a block and increased risk of fracture, or too small a block and inadequate fixation/osseous integration | |
A ruler and bovie may be used to mark oscillating saw cuts; a straight osteotome is used only for release of the bone plug | ||
The graft is passed through the tibial tunnel and fixed in the femur first with a 7 mm × 20 mm titanium interference screw positioned superiorly on the tunnel (to maximize cortical contact of the graft) | ||
Tibial fixation occurs with traction on the graft, with the knee in full extension, with a 9 mm × 20 mm titanium interference screw | ||
HS autograft | Use an open stripper during graft harvesting to ensure the distal gracilis tendon insertion remains intact | Failure to harvest a sufficiently long graft will result in a shortened final construct after folding that may be unsuitable for grafting |
Optimal final graft length after folding is between 6.0 and 6.5 cm | Increased rates of infection have been theorized to be caused by grafts hanging down from their distal insertions and contacting the lower areas of the leg, sterile field, and gown during harvesting | |
Ideal tunnel size may be measured directly from the graft after preparation | ||
QT autograft | The tendon is often more lateral than anticipated, and thus positioning of the bone block cuts should be carefully aligned to create a straight graft | Marking the bone block too lateral will not align with the thickest part of the patella, which is medial |
Osteotomes should be used minimally (only for release of pre-cut bone) or not at all to reduce risk of patellar fracture | Avoid plunging through the patella too deeply to avoid damage to the femoral condylar surfaces | |
Ensure the dissection goes deep enough into the second layer of the quadriceps tendon to avoid harvesting a graft that is too thin | ||
Allograft | Achilles allograft bone block should be sized to 10 mm × 20 mm, and soft-tissue graft shaping should retain uninterrupted fibers | Proper choice of patient population use is crucial to avoid failure in patients at high risk of graft rupture |
A list of critical surgical and rehabilitation principles for optimizing success in various ACLR techniques. ACLR, anterior cruciate ligament reconstruction; ACL, anterior cruciate ligament; BTB, bone-patellar tendon-bone; HS, hamstring; QT, quadriceps tendon.
Optimal graft selection is a critical element of successful ACLR (Table 2). BTB and either single-bundle or double-bundle HS grafts are most frequently used for ACLR (39). Past studies have described the anatomic landscape of the ACL femoral and tibial attachments (Figures 4,5) (41,42). However, attention must also be given to the morphology of the ACL within the joint space. Graft selection which does not resemble the ACL midsubstance structure has been associated with increased risk of graft impingement and increases the risk of ACLR failure (Figure 6) (39,43). Using 3D magnetic resonance imaging (MRI) reconstructions, Thein et al. reported that native ACLs are flatter in the middle with decreased cross-sectional area, and experience decreased impingement, compared to anatomically-oriented BTB ACL grafts (39). In addition, a cadaveric study by Triantafyllidi et al. reported that BTB, semitendinosus tendon, and gracilis tendon grafts have an undersized tibial insertion footprint, compared to the native ACL (43). Thicker ACL grafts have also been reported to disturb the native orientation of the PCL, particularly in the proximal femoral notch (39). Therefore, graft selection with a thin midsubstance, or careful shaping of the graft before implantation is recommended to avoid impingement and decrease the risk of ACLR failure.
Table 2
Techniques | Advantages | Disadvantages | Questions |
---|---|---|---|
BTB autograft | Least amount of graft laxity | Donor site morbidity: risk of anterior knee pain | Can other techniques provide similar success without anterior knee pain? |
Low risk of failure/revision | Most technically challenging autograft technique | ||
Double-sided bone plug | Not advisable for skeletally immature patients | ||
Easily accessible | |||
Consistently reproducible | |||
Well-studied/good evidence | |||
HS autograft | Can be used in skeletally immature patients | Increased rates of graft laxity | How does the small increase in graft laxity differ in patient satisfaction between athletes and non-athletes? |
Easily accessible | Increased rates of infection | ||
Donor site morbidity: risk of HS power loss | |||
QT autograft | Increased length, tensile strength | Donor site morbidity: risk of quadriceps retraction, increased loss of quad strength, increased bleeding | How does long-term follow up compare to other graft choices? |
Decreased anterior knee pain compared to BTB | Long-term evidence not robust | How much quad strength is compromised, and how does this affect athletes? | |
Early study outcomes comparable to BTB | |||
Allograft | No donor-site morbidity | Increased rates of failure compared to autograft | Can allograft failure rate be remedied, so as to eliminate the need for donor-site morbidity? |
Usable in any age population | Longer time to incorporate into bone than autograft | ||
ACL repair | No donor-site morbidity | Higher failure rate compared to autograft, especially BTB, especially age <21 years | How does long-term data compare to ACLR? |
Restoration of native tissue | Long-term evidence not robust | Can ACL repair be improved to match or exceed outcomes of ACLR? | |
LET | Reduces rotational laxity | Additional invasive procedure | Is the risk-to-benefit ratio of LET warranted in elite athletes as an ‘at-risk’ population for graft failure? |
May decrease rates of graft failure when compared to isolated ACLR, especially in at-risk populations | Increased post-operative stiffness |
A comparison of selected autograft, allograft, repair, and augmentation techniques for the ACL. ACL, anterior cruciate ligament; BTB, bone-patellar tendon-bone; HS, hamstring; QT, quadriceps tendon; ACLR, anterior cruciate ligament reconstruction; LET, lateral extraarticular tenodesis.
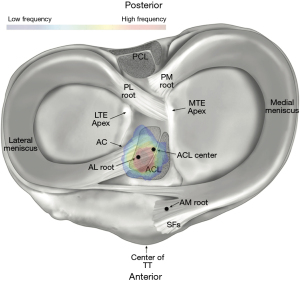
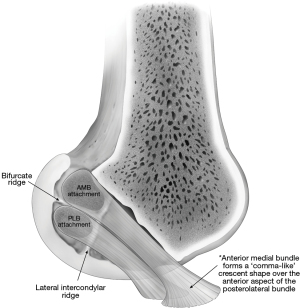
Establishing an optimal preconditioning protocol based on graft type is necessary to produce an ACL graft that is biomechanically equivalent to the native ACL. An in vitro biomechanical study performed by Jaglowski et al. reported that increased-load cyclic and static graft preconditioning protocols resulted in significantly decreased postoperative graft elongation when compared to lower graft preconditioning loads (44). Specifically, use of a static load of 600 N for 20 seconds was found to have the least amount of displacement during simulated rehabilitation, and may be optimal for the clinical setting compared to a 600 N cyclical load. The standard load of 80 N was associated with significantly increased postoperative graft elongation compared to the 600 N static load. Despite this result, the 80 N load remains the standard of care in many institutions (44-46). Additional studies have found that graft preconditioning with a decreased load (~80 N) does not sufficiently precondition the graft and increases the risk for considerable postoperative graft elongation (47-49).
Return to sport following ACLR for high-level athletes requires graft fixation able to withstand enhanced loads on the knee joint during competition. Previous studies have reported the isolated use of interference screws for tibial fixation to be associated with graft slippage and dislodgement, resulting in low ultimate failure loads and the delay of postoperative rehabilitation (50,51). A porcine model biomechanical study by Walsh et al. reported that tibial ACL graft fixation with a combination of retrograde screw and suture button placement had significantly greater failure load than graft fixation with suture button or retrograde screw alone (50). Furthermore, increased graft load strength with usage of both interference screw and a suture-post screw was reported in human patellar tendon grafts (52). Therefore, we assert that the most secure tibial fixation of soft tissue grafts can be achieved with hybrid fixation to maximize graft failure load and decrease the risk of ACLR failure for high-level athletes. For additional surgical pearls and pitfalls for ACLR (see Table 1).
Repair vs. reconstruction
While the idea of a primary repair for ACL injury is appealing, it has met with controversial results in various studies. A promising randomized controlled trial by Murray et al. found patient reported outcomes to be the same or better for bridge-enhanced ACL repair (BEAR) vs. ACLR with HS or BTB autograft (53). However, there are a number of concerning aspects of that study, the first being the control group they used was HS autograft, which the authors of this paper have advocated against being gold standard, especially for high-level athletes. Secondly, the 2-year failure rate for the repair was 14% which was 2.33× higher than the HS group (6%) and significantly higher than published failure rates in BTB population.
Another study proving the effectiveness of primary repair also showed similar subjective outcomes at two years between repair and reconstruction in a randomized controlled trial, but also reported greater necessity of additional surgical procedures in two years of follow up for the repair group (54). Of note, both of these studies used predominantly HS autografts (two patients total had BTB autografts), and it is worth noting that return to higher activity—especially elite level athletics—may differ between BTB autograft and a choice with donor-site morbidity associated with decrease in function, like HS autografts.
Age is another factor to consider regarding ideal candidates for ACL repair. DiFelice reported on 113 patients undergoing primary repair for proximal tears and noted patients under 21 years of age had a failure rate of 37% compared with 4.2% in patients aged 22–35 and 3.5% in those >35 (55). This would suggest repair is a poor option in younger high-level athletes and perhaps athletes in general given athletes in the 21–35 years old age range are likely much more active and high demand than the average patient in the same age group. Treatment of failed repairs is a topic that similarly has a dearth of current literature, however, early reports suggest that surgical management of a failed ACL repair may be notably more complex than a primary ACLR, with some studies reporting 60% of those patients requiring 2-stage ACLR (56). Finally, a review of the subject by Gee et al. rightly pointed out that although short-term outcomes may show promise in carefully considered cohorts, the medium- and long-term outcomes for ACL repair simply do not have enough evidence to support their recommendation at this time (especially in younger patients and athletes) due to higher failure rates of repair (57).
Lateral extra-articular augmentation
Augmentation of ACLR is a topic that continues to be debated. Biomechanical analyses of these reconstructive techniques has demonstrated superior ability to limit internal rotation compared with ACLR alone, and has even demonstrated mild overconstraint in regards to rotation compared with a native ACL state (58,59). Clinical studies have demonstrated similar results to the biomechanic literature. A randomized controlled trial by Getgood et al. found LET augmentation of single-bundle HS tendon ACLR resulted in statistically and clinically significant reductions in graft rupture and persistent rotational laxity (29). A recent systematic review by Na et al. assessed the functional outcomes, stability and complications between patients undergoing isolated ACLR and ACLR augmented with anterolateral extra-articular procedures (AEAP) including anterolateral ligament reconstruction and LET (60). They found that improved pivot shift grades and graft failure rates with AEAP compared with isolated ACLR. It should be noted that higher rates of stiffness were also found in the group undergoing ACLR + LET, suggesting that need for augmentation should be considered and weighed against the risk of additional post-operative stiffness.
Given the notable ability to stabilize and even overconstrain the knee, augmented procedures have gained popularity in at-risk patient populations (61,62). These high-risk populations have traditionally included; adolescent patients (63), revision ACLR (64,65), high grade pivot shift (66), increased posterior slope (67), soft tissue ACLR grafts (29), and knee hyperextension and generalized laxity (63,68). Another population which merits consideration is high-level athletes. Elite athletes have high rates both of primary ACL injury and re-rupture (69). Given these risk factors there has been recent interest in outcomes of augmented procedures in athletes. Borque et al. assessed 455 elite athletes undergoing ACLR or ACLR with LET and found that addition of an LET procedure reduced the rate of re-rupture by 2.8 times (70). More data is needed to evaluate routine use of augmented procedures in athletes who do not possess other high-risk qualities. However, participation in high-level athletics is clearly a risk factor for failure and therefore should factor in to the algorithm of when to use augmented techniques.
Future directions
To conclusively determine optimal graft choice, more high quality long-term comparative studies are required to determine ultimate longevity for different graft choices, and especially for QT autograft, and ACL repair, which we recommend be compared to multiple graft types including BTB autograft. In addition, large randomized, controlled trials may provide high levels of evidence for graft choice, but are difficult to set up. This may be especially useful it determining the utility of augmentation techniques such as LET. Further improvements in recovery for all graft types may also be seen through use of evidence-based post-operative rehabilitation, biologics, or other yet-undiscovered techniques. We strongly advocate attention to post-operative rehabilitation in particular as an under-appreciated aspect of optimal ACLR which merits further high-quality studies.
Conclusions
It is the preference of the corresponding author to use BTB autograft in all patients without a contraindication. It has been our experience that high-level athletes often prefer the trade-off of potential increase in anterior knee pain to the risk of losing performance from donor site morbidity such as in QT or HS autografts, or higher rates of failure and need for revision with use of allografts. However, HS autografts still have an important place in the setting of skeletally immature athletes. While QT autograft is a promising potential alternative to BTB autograft, the lack of high-quality evidence compared to the preponderance of literature evaluating BTB may make many practitioners wary of choosing this technique over one that is so much more highly studied. The increased rate of BTB incorporation into bone along with a high tensile strength and stiffness allow for less risk of failure during rehabilitation and graft maturation, particularly in high-level athletes who may be pressured to push themselves to perform sooner than recommended. When compared to allograft, despite increased risk of pain, the BTB autograft does not have a risk for decreased strength or function, and also lacks the increased risk of failure associated with allografts. It is also easily accessible during surgery, and the technique is easily reproducible with consistency. For elite athletes desirous to return to sport, the BTB autograft is highly recommended, but optimal graft choice will be affected by further considerations including patient age, sport type, level of play, and primary vs. revision ACLR (Table 2).
Acknowledgments
Funding: None.
Footnote
Provenance and Peer Review: This article was commissioned by the Guest Editor (Nicholas N. DePhillipo) for the series “Implications of Graft Choice in ACL Reconstruction” published in Annals of Joint. The article has undergone external peer review.
Peer Review File: Available at https://aoj.amegroups.com/article/view/10.21037/aoj-22-40/prf
Conflicts of Interest: All authors have completed the ICMJE uniform disclosure form (available at https://aoj.amegroups.com/article/view/10.21037/aoj-22-40/coif). The series “Implications of Graft Choice in ACL Reconstruction” was commissioned by the editorial office without any funding or sponsorship. J.K.M. declares that he receives presenter honorarium for the Smith & Nephew MACKIS meeting and support for meeting travel from OSET Meeting, in Boston, MA (9/23-25/2023). N.I.K. declares that he receives $1,500 from Vericel for a Fellows teaching course. R.F.L. declares that he receives research grants from AOSSM, AANA, Ossur, Smith and Nephew, royalties or licenses from Ossur, Smith and Nephew, and Elsevier, and consulting fees from Ossur and Smith and Nephew. He holds patents planned, issued or pending in Ossur, and Smith and Nephew and serves on editorial boards of AJSM, KSSTA, JEO, JKS, and OTSM. The authors have no other conflicts of interest to declare.
Ethical Statement: The authors are accountable for all aspects of the work in ensuring that questions related to the accuracy or integrity of any part of the work are appropriately investigated and resolved. All clinical procedures described in this study were performed in accordance with the ethical standards of the institutional and/or national research committee(s) and with the Helsinki Declaration (as revised in 2013). Written informed consent was obtained from the patients for the publication of this article and accompanying images.
Open Access Statement: This is an Open Access article distributed in accordance with the Creative Commons Attribution-NonCommercial-NoDerivs 4.0 International License (CC BY-NC-ND 4.0), which permits the non-commercial replication and distribution of the article with the strict proviso that no changes or edits are made and the original work is properly cited (including links to both the formal publication through the relevant DOI and the license). See: https://creativecommons.org/licenses/by-nc-nd/4.0/.
References
- Cerulli G, Placella G, Sebastiani E, et al. ACL Reconstruction: Choosing the Graft. Joints 2013;1:18-24. [PubMed]
- Mall NA, Chalmers PN, Moric M, et al. Incidence and trends of anterior cruciate ligament reconstruction in the United States. Am J Sports Med 2014;42:2363-70. [Crossref] [PubMed]
- Hootman JM, Dick R, Agel J. Epidemiology of collegiate injuries for 15 sports: summary and recommendations for injury prevention initiatives. J Athl Train 2007;42:311-9. [PubMed]
- Waldén M, Hägglund M, Magnusson H, et al. ACL injuries in men's professional football: a 15-year prospective study on time trends and return-to-play rates reveals only 65% of players still play at the top level 3 years after ACL rupture. Br J Sports Med 2016;50:744-50. [Crossref] [PubMed]
- Dodson CC, Secrist ES, Bhat SB, et al. Anterior Cruciate Ligament Injuries in National Football League Athletes From 2010 to 2013: A Descriptive Epidemiology Study. Orthop J Sports Med 2016;4:2325967116631949. [Crossref] [PubMed]
- Smith HC, Vacek P, Johnson RJ, et al. Risk factors for anterior cruciate ligament injury: a review of the literature - part 1: neuromuscular and anatomic risk. Sports Health 2012;4:69-78. [Crossref] [PubMed]
- Bradley JP, Klimkiewicz JJ, Rytel MJ, et al. Anterior cruciate ligament injuries in the National Football League: epidemiology and current treatment trends among team physicians. Arthroscopy 2002;18:502-9. [Crossref] [PubMed]
- Dragoo JL, Braun HJ, Durham JL, et al. Incidence and risk factors for injuries to the anterior cruciate ligament in National Collegiate Athletic Association football: data from the 2004-2005 through 2008-2009 National Collegiate Athletic Association Injury Surveillance System. Am J Sports Med 2012;40:990-5. [Crossref] [PubMed]
- Kobayashi H, Kanamura T, Koshida S, et al. Mechanisms of the anterior cruciate ligament injury in sports activities: a twenty-year clinical research of 1,700 athletes. J Sports Sci Med 2010;9:669-75. [PubMed]
- Mai HT, Chun DS, Schneider AD, et al. Performance-Based Outcomes After Anterior Cruciate Ligament Reconstruction in Professional Athletes Differ Between Sports. Am J Sports Med 2017;45:2226-32. [Crossref] [PubMed]
- Barth KA, Lawton CD, Touhey DC, et al. The negative impact of anterior cruciate ligament reconstruction in professional male footballers. Knee 2019;26:142-8. [Crossref] [PubMed]
- Buerba RA, Zaffagnini S, Kuroda R, et al. ACL reconstruction in the professional or elite athlete: state of the art. J ISAKOS 2021;6:226-36. [Crossref] [PubMed]
- Cai J, Xu J, Kang Y, et al. Acceleration of ligamentization and osseointegration processes after anterior cruciate ligament reconstruction with autologous tissue-engineered polyethylene terephthalate graft. Ann Transl Med 2021;9:770. [Crossref] [PubMed]
- Siebold R. Observations on bone tunnel enlargement after double-bundle anterior cruciate ligament reconstruction. Arthroscopy 2007;23:291-8. [Crossref] [PubMed]
- Zhang X, Wu C, Jiang G, et al. The effects of geometry and fiber bundle orientation on the finite element modeling of the anterior cruciate ligament. Annu Int Conf IEEE Eng Med Biol Soc 2008;2008:899-902. [Crossref] [PubMed]
- Erickson BJ, Harris JD, Fillingham YA, et al. Orthopedic Practice Patterns Relating to Anterior Cruciate Ligament Reconstruction in Elite Athletes. Am J Orthop (Belle Mead NJ) 2015;44:E480-5. [PubMed]
- Erickson BJ, Harris JD, Fillingham YA, et al. Anterior cruciate ligament reconstruction practice patterns by NFL and NCAA football team physicians. Arthroscopy 2014;30:731-8. [Crossref] [PubMed]
- McCarty EC, Kraeutler MJ, Langner P, et al. Historical Patterns and Variation in Treatment of Injuries in NFL (National Football League) Players and NCAA (National Collegiate Athletic Association) Division I Football Players. Am J Orthop (Belle Mead NJ) 2016;45:E319-27. [PubMed]
- Farber J, Harris JD, Kolstad K, et al. Treatment of Anterior Cruciate Ligament Injuries by Major League Soccer Team Physicians. Orthop J Sports Med 2014;2:2325967114559892. [Crossref] [PubMed]
- Noyes FR, Butler DL, Grood ES, et al. Biomechanical analysis of human ligament grafts used in knee-ligament repairs and reconstructions. J Bone Joint Surg Am 1984;66:344-52. [Crossref] [PubMed]
- Freedman KB, D'Amato MJ, Nedeff DD, et al. Arthroscopic anterior cruciate ligament reconstruction: a metaanalysis comparing patellar tendon and hamstring tendon autografts. Am J Sports Med 2003;31:2-11. [Crossref] [PubMed]
- Li S, Chen Y, Lin Z, et al. A systematic review of randomized controlled clinical trials comparing hamstring autografts versus bone-patellar tendon-bone autografts for the reconstruction of the anterior cruciate ligament. Arch Orthop Trauma Surg 2012;132:1287-97. [Crossref] [PubMed]
- Gifstad T, Foss OA, Engebretsen L, et al. Lower risk of revision with patellar tendon autografts compared with hamstring autografts: a registry study based on 45,998 primary ACL reconstructions in Scandinavia. Am J Sports Med 2014;42:2319-28. [Crossref] [PubMed]
- Almekinders LC, Moore T, Freedman D, et al. Post-operative problems following anterior cruciate ligament reconstruction. Knee Surg Sports Traumatol Arthrosc 1995;3:78-82. [Crossref] [PubMed]
- Mohtadi NG, Chan DS, Dainty KN, et al. Patellar tendon versus hamstring tendon autograft for anterior cruciate ligament rupture in adults. Cochrane Database Syst Rev 2011;2011:CD005960. [Crossref] [PubMed]
- Brown CH, Steiner ME, Carson EW. The Use of Hamstring Tendons For Anterior Cruciate Ligament Reconstruction: Technique and Results. Clin Sports Med 1993;12:723-56. [Crossref] [PubMed]
- Fabricant PD, Jones KJ, Delos D, et al. Reconstruction of the anterior cruciate ligament in the skeletally immature athlete: a review of current concepts: AAOS exhibit selection. J Bone Joint Surg Am 2013;95:e28. [Crossref] [PubMed]
- Corry IS, Webb JM, Clingeleffer AJ, et al. Arthroscopic Reconstruction of the Anterior Cruciate Ligament. American Journal of Sports Medicine 1999;27:444-54. [Crossref] [PubMed]
- Getgood AMJ, Bryant DM, Litchfield R, et al. Lateral Extra-articular Tenodesis Reduces Failure of Hamstring Tendon Autograft Anterior Cruciate Ligament Reconstruction: 2-Year Outcomes From the STABILITY Study Randomized Clinical Trial. Am J Sports Med 2020;48:285-97. [Crossref] [PubMed]
- Diermeier T, Tisherman R, Hughes J, et al. Quadriceps tendon anterior cruciate ligament reconstruction. Knee Surg Sports Traumatol Arthrosc 2020;28:2644-56. [Crossref] [PubMed]
- Han HS, Seong SC, Lee S, et al. Anterior cruciate ligament reconstruction: quadriceps versus patellar autograft. Clin Orthop Relat Res 2008;466:198-204. [Crossref] [PubMed]
- Maletis GB, Inacio MC, Reynolds S, et al. Incidence of postoperative anterior cruciate ligament reconstruction infections: graft choice makes a difference. Am J Sports Med 2013;41:1780-5. [Crossref] [PubMed]
- Slone HS, Romine SE, Premkumar A, et al. Quadriceps tendon autograft for anterior cruciate ligament reconstruction: a comprehensive review of current literature and systematic review of clinical results. Arthroscopy 2015;31:541-54. [Crossref] [PubMed]
- Lund B, Nielsen T, Faunø P, et al. Is quadriceps tendon a better graft choice than patellar tendon? a prospective randomized study. Arthroscopy 2014;30:593-8. [Crossref] [PubMed]
- Kaeding CC, Aros B, Pedroza A, et al. Allograft Versus Autograft Anterior Cruciate Ligament Reconstruction: Predictors of Failure From a MOON Prospective Longitudinal Cohort. Sports Health 2011;3:73-81. [Crossref] [PubMed]
- Wasserstein D, Sheth U, Cabrera A, et al. A Systematic Review of Failed Anterior Cruciate Ligament Reconstruction With Autograft Compared With Allograft in Young Patients. Sports Health 2015;7:207-16. [Crossref] [PubMed]
- Spindler KP, Huston LJ, Wright RW, et al. The prognosis and predictors of sports function and activity at minimum 6 years after anterior cruciate ligament reconstruction: a population cohort study. Am J Sports Med 2011;39:348-59. [Crossref] [PubMed]
- Vyas D, Rabuck SJ, Harner CD. Allograft anterior cruciate ligament reconstruction: indications, techniques, and outcomes. J Orthop Sports Phys Ther 2012;42:196-207. [Crossref] [PubMed]
- Thein R, Spitzer E, Doyle J, et al. The ACL Graft Has Different Cross-sectional Dimensions Compared With the Native ACL: Implications for Graft Impingement. Am J Sports Med 2016;44:2097-105. [Crossref] [PubMed]
- LaPrade CM, Ellman MB, Rasmussen MT, et al. Anatomy of the anterior root attachments of the medial and lateral menisci: a quantitative analysis. Am J Sports Med 2014;42:2386-92. [Crossref] [PubMed]
- Ziegler CG, Pietrini SD, Westerhaus BD, et al. Arthroscopically pertinent landmarks for tunnel positioning in single-bundle and double-bundle anterior cruciate ligament reconstructions. Am J Sports Med 2011;39:743-52. [Crossref] [PubMed]
- Girgis FG, Marshall JL, Monajem A. The cruciate ligaments of the knee joint. Anatomical, functional and experimental analysis. Clin Orthop Relat Res 1975;216-31. [Crossref] [PubMed]
- Triantafyllidi E, Paschos NK, Goussia A, et al. The shape and the thickness of the anterior cruciate ligament along its length in relation to the posterior cruciate ligament: a cadaveric study. Arthroscopy 2013;29:1963-73. [Crossref] [PubMed]
- Jaglowski JR, Williams BT, Turnbull TL, et al. High-load preconditioning of soft tissue grafts: an in vitro biomechanical bovine tendon model. Knee Surg Sports Traumatol Arthrosc 2016;24:895-902. [Crossref] [PubMed]
- Cunningham R, West JR, Greis PE, et al. A survey of the tension applied to a doubled hamstring tendon graft for reconstruction of the anterior cruciate ligament. Arthroscopy 2002;18:983-8. [Crossref] [PubMed]
- Yasuda K, Tsujino J, Tanabe Y, et al. Effects of initial graft tension on clinical outcome after anterior cruciate ligament reconstruction. Autogenous doubled hamstring tendons connected in series with polyester tapes. Am J Sports Med 1997;25:99-106. [Crossref] [PubMed]
- Boylan D, Greis PE, West JR, et al. Effects of initial graft tension on knee stability after anterior cruciate ligament reconstruction using hamstring tendons: a cadaver study. Arthroscopy 2003;19:700-5. [Crossref] [PubMed]
- Nurmi JT, Kannus P, Sievänen H, et al. Interference screw fixation of soft tissue grafts in anterior cruciate ligament reconstruction: part 2: effect of preconditioning on graft tension during and after screw insertion. Am J Sports Med 2004;32:418-24. [Crossref] [PubMed]
- Labs K, Perka C, Schneider F. The biological and biomechanical effect of different graft tensioning in anterior cruciate ligament reconstruction: an experimental study. Arch Orthop Trauma Surg 2002;122:193-9. [Crossref] [PubMed]
- Walsh MP, Wijdicks CA, Parker JB, et al. A comparison between a retrograde interference screw, suture button, and combined fixation on the tibial side in an all-inside anterior cruciate ligament reconstruction: a biomechanical study in a porcine model. Am J Sports Med 2009;37:160-7. [Crossref] [PubMed]
- Chang HC, Nyland J, Nawab A, et al. Biomechanical comparison of the bioabsorbable RetroScrew system, BioScrew XtraLok with stress equalization tensioner, and 35-mm Delta Screws for tibialis anterior graft-tibial tunnel fixation in porcine tibiae. Am J Sports Med 2005;33:1057-64. [Crossref] [PubMed]
- Steiner ME, Hecker AT, Brown CH Jr, et al. Anterior cruciate ligament graft fixation. Comparison of hamstring and patellar tendon grafts. Am J Sports Med 1994;22:240-7. [Crossref] [PubMed]
- Murray MM, Fleming BC, Badger GJ, et al. Bridge-Enhanced Anterior Cruciate Ligament Repair Is Not Inferior to Autograft Anterior Cruciate Ligament Reconstruction at 2 Years: Results of a Prospective Randomized Clinical Trial. Am J Sports Med 2020;48:1305-15. [Crossref] [PubMed]
- Hoogeslag RAG, Brouwer RW, Boer BC, et al. Acute Anterior Cruciate Ligament Rupture: Repair or Reconstruction? Two-Year Results of a Randomized Controlled Clinical Trial. Am J Sports Med 2019;47:567-77. [Crossref] [PubMed]
- Vermeijden HD, Yang XA, van der List JP, et al. Role of Age on Success of Arthroscopic Primary Repair of Proximal Anterior Cruciate Ligament Tears. Arthroscopy 2021;37:1194-201. [Crossref] [PubMed]
- Cristiani R, Mouton C, Siboni R, et al. Failure of primary ACL repair with dynamic intraligamentary stabilization may result in a high risk of two-stage ACL reconstruction: a case series of ten patients. J Exp Orthop 2022;9:79. [Crossref] [PubMed]
- Gee MSM, Peterson CDR, Zhou ML, et al. Anterior Cruciate Ligament Repair: Historical Perspective, Indications, Techniques, and Outcomes. J Am Acad Orthop Surg 2020;28:963-71. [Crossref] [PubMed]
- Geeslin AG, Moatshe G, Chahla J, et al. Anterolateral Knee Extra-articular Stabilizers: A Robotic Study Comparing Anterolateral Ligament Reconstruction and Modified Lemaire Lateral Extra-articular Tenodesis. Am J Sports Med 2018;46:607-16. [Crossref] [PubMed]
- Slette EL, Mikula JD, Schon JM, et al. Biomechanical Results of Lateral Extra-articular Tenodesis Procedures of the Knee: A Systematic Review. Arthroscopy 2016;32:2592-611. [Crossref] [PubMed]
- Na BR, Kwak WK, Seo HY, et al. Clinical Outcomes of Anterolateral Ligament Reconstruction or Lateral Extra-articular Tenodesis Combined With Primary ACL Reconstruction: A Systematic Review With Meta-analysis. Orthop J Sports Med 2021;9:23259671211023099. [Crossref] [PubMed]
- Geeslin AG, Chahla J, LaPrade RF. Combined Anterior Cruciate Ligament and Lateral Extra-Articular Reconstruction. Arthroscopy 2022;38:2600-1. [Crossref] [PubMed]
- Ziegler CG, DePhillipo NN, Kennedy MI, et al. Beighton Score, Tibial Slope, Tibial Subluxation, Quadriceps Circumference Difference, and Family History Are Risk Factors for Anterior Cruciate Ligament Graft Failure: A Retrospective Comparison of Primary and Revision Anterior Cruciate Ligament Reconstructions. Arthroscopy 2021;37:195-205. [Crossref] [PubMed]
- Guarino A, Farinelli L, Iacono V, et al. Lateral extra-articular tenodesis and anterior cruciate ligament reconstruction in young patients: clinical results and return to sport. Orthop Rev (Pavia) 2022;14:33696. [Crossref] [PubMed]
- Madhan AS, Ganley TJ, McKay SD, et al. Trends in Anterolateral Ligament Reconstruction and Lateral Extra-articular Tenodesis With ACL Reconstruction in Children and Adolescents. Orthop J Sports Med 2022;10:23259671221088049. [Crossref] [PubMed]
- Alm L, Drenck TC, Frosch KH, et al. Lateral extra-articular tenodesis in patients with revision anterior cruciate ligament (ACL) reconstruction and high-grade anterior knee instability. Knee 2020;27:1451-7. [Crossref] [PubMed]
- Akmese R, Ovali SA, Celebi MM, et al. A Surgical Algorithm According to Pivot-Shift Grade in Patients With ACL Injury: A Prospective Clinical and Radiological Evaluation. Orthop J Sports Med 2021;9:23259671211025494. [Crossref] [PubMed]
- Akoto R, Alm L, Drenck TC, et al. Slope-Correction Osteotomy with Lateral Extra-articular Tenodesis and Revision Anterior Cruciate Ligament Reconstruction Is Highly Effective in Treating High-Grade Anterior Knee Laxity. Am J Sports Med 2020;48:3478-85. [Crossref] [PubMed]
- Mahmoud A, Torbey S, Honeywill C, et al. Lateral Extra-Articular Tenodesis Combined With Anterior Cruciate Ligament Reconstruction Is Effective in Knees With Additional Features of Lateral, Hyperextension, or Increased Rotational Laxity: A Matched Cohort Study. Arthroscopy 2022;38:119-24. [Crossref] [PubMed]
- Gans I, Retzky JS, Jones LC, et al. Epidemiology of Recurrent Anterior Cruciate Ligament Injuries in National Collegiate Athletic Association Sports: The Injury Surveillance Program, 2004-2014. Orthop J Sports Med 2018;6:2325967118777823. [Crossref] [PubMed]
- Borque KA, Jones M, Laughlin MS, et al. Effect of Lateral Extra-articular Tenodesis on the Rate of Revision Anterior Cruciate Ligament Reconstruction in Elite Athletes. Am J Sports Med 2022;50:3487-92. [Crossref] [PubMed]
Cite this article as: Homan MD, Braaten JA, Banovetz MT, Monson JK, Kennedy NI, LaPrade RF. Principles for optimizing anterior cruciate ligament reconstruction outcomes in elite athletes: a review of current techniques. Ann Joint 2024;9:19.